Accuracy of maximal expiratory flow-volume curve curvilinearity and fractional exhaled nitric oxide for detection of children with atopic asthma
Article information
Abstract
Purpose
Airway pathology in children with atopic asthma can be reflected by the concave shape of the maximal expiratory flow-volume (MEFV) curve and high fractional exhaled nitric oxide (FeNO) values. We evaluated the capacity of the curvilinearity of the MEFV curve, FeNO, and their combination to distinguish subjects with atopic asthma from healthy individuals.
Methods
FeNO and angle β, which characterizes the general configuration of the MEFV curve, were determined in 119 steroid-naïve individuals with atopic asthma aged 8 to 16 years, and in 92 age-matched healthy controls. Receiver operating characteristic (ROC) curve analyses were performed to determine the cutoff points of FeNO and angle β that provided the best combination of sensitivity and specificity for asthma detection.
Results
Asthmatic patients had a significantly smaller angle β and higher FeNO compared with healthy controls (both, P<0.001). For asthma detection, the best cutoff values of angle β and FeNO were observed at 189.3° and 22 parts per billion, respectively. The area under the ROC curve for the combination of angle β and FeNO improved to 0.91 (95% confidence interval [CI], 0.87–0.95) from 0.80 (95% CI, 0.75–0.86; P<0.001) for angle β alone and 0.86 (95% CI, 0.82–0.91; P=0.002) for FeNO alone. In addition, the combination enhanced sensitivity with no significant decrease in specificity.
Conclusion
These data suggest that the combined use of the curvilinearity of the MEFV curve and FeNO is a useful tool to differentiate between children with and without atopic asthma.
Introduction
Asthma diagnosis is primarily based on a typical history and a clinical examination during an acute episode. Although good responses to treatment in asthmatic patients with episodic symptoms support a diagnosis of asthma, spirometry to demonstrate airflow obstruction has been recommended for patients in whom the diagnosis of asthma is under consideration12). Airflow obstruction is indicated by a reduction in the values of both forced expiratory volume in 1 second (FEV1) and FEV1/forced vital capacity (FVC) relative to reference or predicted values. In addition, slowing of the expiratory flow resulting from airflow obstruction induces a concave shape in the maximal expiratory flow-volume (MEFV) curve during forced exhalation3). As determined in previous studies456), a concave MEFV curve is a sensitive test of airflow limitation and can also be detected in subjects who have normal values of traditional lung function parameters. Previously, Kapp et al.7) defined a marker, angle β, to characterize the shape of the MEFV curve and found that asthmatic patients had a significantly lower angle β than healthy individuals. More importantly, they also reported that angle β had less within- and between-day variability than other lung function parameters, including FEV1. Therefore, it is plausible that angle β could provide a complementary tool to other identification methods of asthmatic patients among subjects with respiratory symptoms.
Measurement of fractional exhaled nitric oxide (FeNO) is a quantitative, noninvasive, simple, and safe method used to determine eosinophilic inflammation of the airway8). FeNO elevation in subjects with asthma helps establish the correct asthma diagnosis. The accuracy of FeNO measurements in previous clinic-based studies appears to be acceptable for discriminating asthma from nonasthma conditions9101112). However, because FeNO levels are not increased in nonatopic asthmatic patients131415), FeNO appears to be valuable only in the diagnosis of atopic asthma.
While the use of FeNO has been recommend for the diagnosis of eosinophilic airway inflammation15), there has been a shortage of information on the role of angle β in the diagnosis of atopic asthma. The aim of this study was to determine the angle β cutoff point that best differentiates children with asthma from healthy children and evaluate whether the combined use of angle β and FeNO enhances discriminatory accuracy.
Materials and methods
1. Subjects
This study included children aged 8 to 16 years with and without asthma recruited between July 2015 and December 2016. Asthma was defined based on the Global Initiative for Asthma guidelines2), which include characteristic episodic respiratory symptoms and reversible airflow obstruction (documentation of variability of FEV1 by 12% in response to inhaled β2-agonist). All of asthmatic patients were classified as having intermittent or mild persistent asthma based on the guidelines from the National Asthma Education and Prevention Program1) and found to be sensitized to more than one aeroallergen in skin prick tests. The mean duration of asthma was 3.9 years. The patients were using no asthma medications except short-acting bronchodilators, which were withheld for at least 4 hours prior to testing. No regular treatment with controller medications such as inhaled corticosteroid (ICS) and leukotriene antagonists had been used within 8 weeks of enrollment. Healthy control subjects were selected from children who visited a pediatric center and a random sample of nonatopic children without a history of asthma or related respiratory symptoms as defined by the International Study of Asthma and Allergies in Childhood questionnaire. All subjects had been free of respiratory infections for at least 4 weeks.
The Ethics Committee of Chungbuk National University Hospital Institutional Review Board approved this study (CBNUH IRB No. 2011-05-029) and written informed consent was obtained from all subjects and their parents.
2. Protocol
FeNO and lung function were measured on the same day in all patients. FeNO measurement was performed first, followed by spirometry. The measurements were performed by the same operators who were blinded to patient histories and diagnoses. Chest and sinus radiography were performed at the discretion of the investigators.
3. FeNO measurement
FeNO was measured by an NO analyzer with electrochemical sensors (NIOX MINO, Aerocrine AB, Solna, Sweden), according to American Thoracic Society (ATS)/European Respiratory Society (ERS) guidelines16), and expressed as parts per billion (ppb). The children were instructed to avoid eating, drinking, and strenuous exercise 2 hours before FeNO measurements. After inhalation of ambient air through a nitric oxide scrubber to total lung capacity, subjects then exhaled against expiratory resistance to exclude nasal air. Exhalation times were 10 seconds with a 2-minute analysis period. Repeated exhalations (2 values that agreed within 5% or 3 that agreed within 10%) were performed without a nose clip at a constant flow rate of 50 mL/sec.
4. Pulmonary function testing
Lung function tests were performed with a spirometer (Vmax SensorMedics, Yorba Linda, CA, USA) in accordance with ATS/ERS recommendations17). Lung function tests were performed between 2 PM and 5 PM in the afternoon. The following variables were obtained from the best of 3 reproducible forced expiratory maneuvers: forced vital capacity (FVC), forced expiratory volume in 1 second (FEV1), FEV1/FVC ratio, forced expiratory flow between 25% and 75% of vital capacity (FEF25%–75%), forced expiratory flow at 50% of vital capacity (FEF50%), and peak expiratory flow (PEF). Percent predicted values were calculated according to the Third National Health and Nutrition Examination Survey (NHANES III)18). The concave shape of the MEFV curve was defined as a curve with a scoop between the peak of the curve and the endpoint of expiration (Fig. 1). The curvilinearity of the MEFV curve was determined by angle β which was calculated based on Kapp's method7) as follows:

Schematic illustrations of the convex (A), linear (B), and concave (C) shapes of a maximum expiratory flow-volume curve. PEF, peak expiratory flow; FEF, forced expiratory flow.
All tan−1 values were calculated in degrees.
5. Statistical analysis
Descriptive data for demographic and clinical characteristics of study subjects are presented as mean and 95% confidence interval (CI) for continuous variables and as frequencies for categorical variables. Normality test was performed on the all numeric variables using Shapiro-Wilk test. Total serum IgE, blood eosinophil count, and FeNO values were logarithmically transformed to assume a normal distribution and expressed as geometric means with 95% CI. A t-test was used to compare means. The chi-square test was used to compare frequencies of categorical variables. Receiver operating characteristic curve (ROC) analysis was performed to determine the best cutoff threshold for angle β, FeNO, and the combination of angle β and FeNO. The combination of angle β and FeNO was established using a logistic regression model and its prediction probability was used as a discriminating indicator. The area under the ROC curve (AUC), sensitivity, specificity, positive predictive values (PPVs), and negative predictive values (NPVs) were obtained to assess discriminating accuracy. The DeLong test was used to compare AUCs19). A P value of <0.05 was considered significant. All statistical analyses were performed using IBM SPSS Statistics ver. 23.0 (IBM Co., Armonk, NY, USA).
Results
Two hundred eleven subjects were recruited. This study population comprised 119 individuals with atopic asthma and 92 healthy controls. The characteristics of individuals with and without atopic asthma are presented in Table 1. There was no difference in age, sex distribution, anthropometric values, or exposure to parental smoking between groups. However, geometric mean (GM) total serum IgE levels and eosinophil numbers were significantly higher in atopic asthmatic patients than in healthy control subjects. As expected, lung functions were lower in the atopic asthma group than in the control group. There was a significant difference in mean angle β between patients in the atopic asthma (184.8°; 95% CI, 181.3–188.3) and control (204.8°; 95% CI, 202.0–207.6) groups (P<0.001). The GM FeNO in atopic asthmatic patients was 25.0 ppb (95% CI, 22.4 –27.9), which was expectedly higher than 10.9 ppb (95% CI, 10.0–11.9) in healthy controls (P<0.001).
We constructed ROC curves to determine the sensitivity and specificity of angle β and FeNO for the detection of atopic asthma (Fig. 2). The sensitivity, specificity, positive and NPVs for best cutoff, angle β, and FeNO are listed in Table 2. The AUCs for angle β and FeNO were 0.80 (95% CI, 0.75–0.86) and 0.86 (95% CI, 0.82–0.91), respectively. The highest sums of the sensitivity and specificity for the cutoff values of angle β and FeNO were observed at 189.3° and 22 ppb, respectively.

Receiver operator characteristic curves indicating the sensitivity and specificity of angle β, fractional exhaled nitric oxide (FeNO), and their combination for the detection of atopic asthma.
We next compared the combination of angle β and FeNO to each measurement alone in discriminating atopic asthmatic patients from healthy controls. As shown in Table 2, the AUC for the combination of angle β and FeNO improved to 0.91 (95% CI, 0.87–0.95) from those for angle β alone and FeNO alone. The differences between the AUCs were statistically significant when tested using the DeLong method (Table 3). In addition, compared to FeNO alone, the combination of angle β and FeNO improved sensitivity and NPV with only a small decrease in specificity and PPV, while it improved sensitivity, specificity, PPV, and NPV for angle β alone (Table 2).

Comparisons of the areas under the receiver operating characteristic curve for the detection of atopic asthma
The distribution of atopic asthmatic patients according to the levels of angle β and FeNO is shown in Fig. 3. When these patients were dichotomized into large and small angle β groups based on the best cutoff value of angle β (≥189.3 degrees for angle βlarge vs. <189.3 degrees for angle βsmall), 53 (45%) and 66 patients (55%) belonged to the angle βlarge and angle βsmall groups, respectively. The mean angle β of the angle βlarge and angle βsmall groups was 201.6° (95% CI, 199.5–203.8) and 171.3° (95% CI, 167.6–174.9), respectively. Although the GM FeNO in the angle βsmall group (29.8 ppb; 95% CI, 26.2–34.0) was higher than that in the angle βlarge group (25.0 ppb; 95% CI, 21.4–29.2), this difference was not significant (P=0.083).
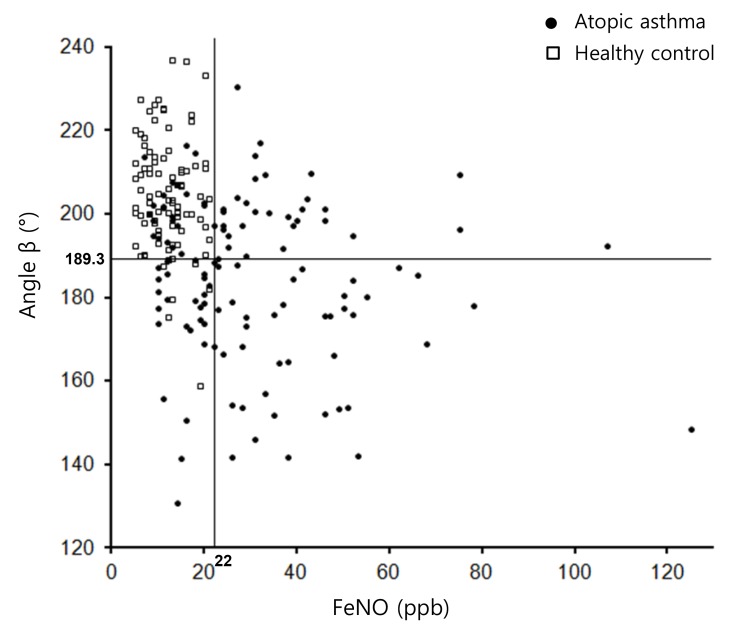
The distribution of patients according the values of angle β and fractional exhaled nitric oxide (FeNO). Lines indicate the best cutoff values of angle β and FeNO for the detection of atopic asthma. ppb, parts per billion.
Atopic asthmatic patients were also divided into groups of high and low FeNO based on the best FeNO cutoff value (>22 ppb for FeNOhigh vs. ≤22 ppb for FeNOlow). An FeNO level of 22 ppb or higher was observed in 86 patients (72%), while 33 patients (28%) had an FeNO level lower than 22 ppb. GM FeNO in the FeNOhigh and FeNOlow groups was 35.8 ppb (95% CI, 33.0–38.8) and 13.9 ppb (95% CI, 12.6–15.4), respectively. There was no statistical difference in the mean angle β between the FeNOhigh (183.3°; 95% CI, 178.9–187.6) and FeNOlow groups (188.7°; 95% CI, 182.8–194.6; P=0.172).
Discussion
In this study, angle β quantitation of the curvilinearity of the MEFV curve and FeNO were shown to differ significantly between atopic asthmatic patients and healthy individuals. In an ROC curve analysis, we documented that the discriminating potential of these 2 markers for atopic asthma is robust. However, the discriminatory capacity of angle β and FeNO produced relatively low sensitivity due to a considerable amount of overlap in these markers between atopic asthmatic patients and healthy controls. To overcome this drawback, we used angle β and FeNO in combination and found that it was a more accurate discriminator of atopic asthma than either marker alone.
The importance of detecting concavity in the MEFV curve was described by the ATS/ERS guidelines as the earliest change associated with airflow obstruction in small airways3). A concave-shaped MEFV curve during the first visit has been described as a predictor of asthma among school-aged children420). In addition, children with wheezing disorders had lower values of angle β than healthy children21). We also found that atopic asthmatic children had a lower angle β than healthy controls. Given the rarity of other chronic diseases with visible concavity of the MEFV curve, such as cystic fibrosis and chronic obstructive pulmonary disease, these findings strongly suggest that angle β may help differentiate atopic asthmatic patients from healthy children. However, there was a disadvantage in using angle β to differentiate atopic asthma, because only 55% of the atopic asthmatic patients showed an angle β lower than the best cut-off value that distinguished atopic asthmatic and nonasthmatic patients. As a result, 45% of atopic asthmatic patients had an angle β that was not very different from that of healthy controls, indicating the rather poor discriminatory capacity of this marker.
FeNO is primarily increased in atopic asthma131415), which contrasts with normal or near-normal FeNO in nonatopic asthma. For this reason, we limited the asthma group to atopic children. Thus, FeNO observed in our asthmatic patients might be higher than in a population of asthmatic children that includes nonatopic subjects. However, we excluded atopic individuals from the nonasthma group, which might contribute to lower FeNO in this group. As expected, GM FeNO in the nonasthma group was only 10.9 ppb, which was close to those of healthy individuals reported in previous studies using the same method22232425). Due to the significant difference in FeNO between the atopic asthma and nonasthma groups, FeNO measurements provided a high specificity for detection of asthma in this study. However, the sensitivity was relatively low, indicating the limitation of FeNO measurements. This result came from the inclusion of the false-negative cases, because 28% of asthmatic patients had a FeNO concentration of 21 ppb or lower.
Although angle β and FeNO represent a potentially useful tool for the detection of atopic asthma, sensitivity was not satisfactory, as described above. To improve upon the results obtained with each measurement, we evaluated whether their combined determination enhanced their discriminating accuracy. When the combination of the 2 measurements was considered, a significant increase in AUC was observed. In particular, sensitivity increased with no significant sacrifice of specificity. Thus, the combined use of the two markers improved the discriminatory capacity for atopic asthma detection. Moreover, the lack of a statistical difference in FeNO between subjects with large and small angle β, and in angle β between subjects with high and low FeNO, provided the rationale for the combination of the 2 markers.
Subtle variations in the interpretation of an individual patient's signs and symptoms may greatly influence asthma diagnosis. For this reason, more objective evidence is needed to support an asthma diagnosis. Considering that spirometric evaluation and FeNO measurements are relatively simple to perform in nearly all school-age children26), the combined use of angle β and FeNO can become a practical tool that may help physicians detect children with atopic asthma, especially when the child has only mild clinical symptoms.
Several limitations of this study must be addressed. First, our nonasthmatic population consisted of normal prescreened healthy control subjects. Therefore, our cohorts might not reflect the real-world situation faced by the clinician in practice, where asthmatic children must be differentiated from a population of children with a variety of respiratory symptoms. Second, because angle β and FeNO were analyzed in a cross-sectional fashion, our study could not provide interreading variability or true profiles of these markers. For this reason, our findings cannot be generalized to other populations.
In summary, although normal angle β and FeNO values did not exclude the diagnosis of atopic asthma, small angle β and high FeNO could discriminate asthmatic from nonasthmatic patients. In addition, the combined use of both markers could provide diagnostic information superior to either alone. Therefore, the atopic asthma-related changes of angle β and FeNO allow physicians to use these markers to detect children with probable asthma.
Notes
Conflicts of interest: No potential conflict of interest relevant to this article was reported.