The use of extracorporeal membrane oxygenation in children with acute fulminant myocarditis
Article information
Abstract
Acute fulminant myocarditis (AFM) occurs as an inflammatory response to an initial myocardial insult. Its rapid and deadly progression calls for prompt diagnosis with aggressive treatment measures. The demonstration of its excellent recovery potential has led to increasing use of mechanical circulatory support, especially extracorporeal membrane oxygenation (ECMO). Arrhythmias, organ failure, elevated cardiac biomarkers, and decreased ventricular function at presentation predict requirement for ECMO. In these patients, ECMO should be considered earlier as the clinical course of AFM can be unpredictable and can lead to rapid haemodynamic collapse. Key uncertainties that clinicians face when managing children with AFM such as timing of initiation of ECMO and left ventricular decompression need further investigation.
Introduction
Myocarditis is an inflammatory disease caused by an initial insult to the heart which is followed by lymphocytic inflammation of the myocardium [1]. The clinical course of myocarditis ranges from a mild to severe form of heart failure. The severe form of myocarditis that necessitates aggressive and timely treatment to prevent rapid haemodynamic collapse is termed acute fulminant myocarditis (AFM). In certain circumstances, the only way to prevent imminent death is to support the patient on mechanical circulatory support (MCS). Extracorporeal membrane oxygenation (ECMO) is one form of MCS.
ECMO, in the veno-arterial (VA) configuration, is increasingly being used in both pediatric and adult populations for refractory cardiogenic shock [2]. VA-ECMO (simplified as ECMO in this manuscript from here on) represents a modality where blood is drained from a large vein, oxygenated and returned to the circulation through a large artery (Fig. 1).
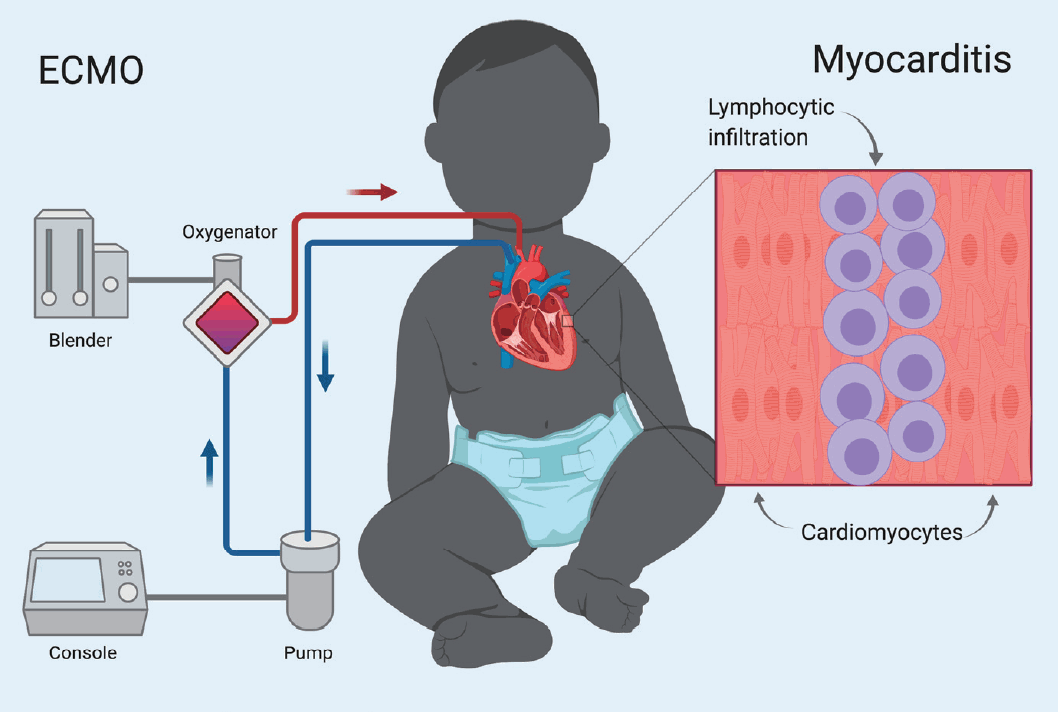
Treatment of acute fulminant myocarditis with veno-arterial extracorporeal membrane oxygenation (VA-ECMO) in children acute fulminant myocarditis manifests as lymphocytic infiltration in the myocardium. Rapid, severe disease course, and excellent long-term outcomes justify early use of aggressive management such as ECMO.
Given ECMO is only life-sustaining in these rapidly deteriorating patients, a thorough understanding of when and how it can be employed is prudent. This comprehensive review has a detailed account of the characteristics of AFM, the clinical scenarios where ECMO might be employed, basic management strategies of a patient on ECMO, further management options and outcomes.
Prevalence of AFM in children
AFM accounts for 10% to 38% of all cases of acute myocarditis [3-5]. Importantly, AFM may account for approximately 10%–20% of sudden and unexplained deaths in children [6-13]. In a United States study, there was a bimodal age distribution with peaks in infancy and mid-teenage years, with a mean age of 9 years [14]. Interestingly, about 60% of patients with AFM are male, a phenomenon observed perhaps due to pubertal hormonal changes in boys [15]. However, females seem to have worse ventricular function at admission, higher mortality, higher need for cardiac transplants and readmissions to hospital within the first year [16]. In a Japanese survey, only 12% had remarkable comorbidities or past medical history, of which most prevalent was congenital heart disease [13].
1. Aetiology, pathophysiology, and disease progression
The aetiology of myocarditis is diverse, as it can be caused by infectious, autoimmune, or toxic agents. In developed countries, the predominant cause of pediatric acute myocarditis is viral infections [17].
The pathophysiological sequence of myocyte injury in acute myocarditis occurs in 2 distinct phases [18]. During the initial stage, a pathogen causes cell injury, lysis and myocardial necrosis, leading to ventricular dysfunction, heart failure, and arrhythmias. This triggers an inflammatory reaction resulting in lymphocyte infiltration.
Intravenous immunoglobulin (IVIG) has been used to limit this immune response, though there are no convincing data as yet demonstrating a clear survival benefit [19,20]. Even if IVIG were beneficial, the immune-modulatory effects are not immediate. Despite limited and inconclusive evidence, IVIG is still considered a potential therapeutic strategy. Proponents observe that the associated risks are relatively small when compared to the morbidity and mortality from AFM [16,17]. Similarly, steroids have been employed with not much reported benefit [21].
Children with AFM are some of the sickest patients. In these patients, hemodynamic compromise can be sudden. Consequently, early diagnosis and prompt management with aggressive therapies can be lifesaving.
2. Medical treatments for hemodynamic stabilization
The aims of therapeutic interventions in a patient with AFM is to augment cardiac output, to stabilize hemodynamic variables, and to optimise oxygen delivery-demand mismatch. Positive-pressure ventilation assists by reducing the work of breathing and left ventricular afterload. Although intubation and sedation decrease metabolic demands, it must be done with caution, since these patients are at high risk of severe hemodynamic deterioration and cardiac arrest during intubation [22]. Cautious fluid resuscitation and intravenous inotropic agents are the mainstay of treatment [17]. Some of the predictors of a fulminant myocarditis course is outlined in Table 1.
Extracorporeal membrane oxygenation
1. Applications
When a patient is not adequately perfused on maximal medical therapy, ECMO offers a sensible escalation strategy. In these patients, ECMO might be employed as a bridge to decision, bridge to recovery (BTR), or bridge to transplant (BTT). A summary of the main indications for ECMO is presented in Table 2. Retrospective studies that have investigated the use of ECMO in pediatric AFM in the last decade are also reviewed in Table 3.
ECMO is not a definitive treatment for myocarditis as it does not reverse native cardiac dysfunction. But, ECMO can support whole-body perfusion while cardiac function recovers. Although, the time-point when a patient is placed on ECMO varies between centres, there is general consensus that ECMO must be instituted before end-organ dysfunction ensues. Given the decision to place on ECMO and the timing are vitally important for the survival and long-term outcome of the patients, proactive multidisciplinary discussions about goals and strategies should take place in the early phases of illness.
2. Cannulation
Peripheral cannulation is the preferred approach for children with AFM. The variables to consider in the choice of location are the size of the ECMO cannulae relative to the vessel size and the condition of vessels (i.e., previously accessed/thrombosed). Femoral vessels are not well developed before children start walking. Hence if chosen as a site, there is an increased the risk of limb ischemia and gangrene while access itself may be harder. Crucially, the desired blood flows may not be achieved [32,33]. Consequently, contrary to adult ECMO, the cannulation for neonates, infants, and small children is primarily in the neck (drained from right internal jugular vein and perfused to right common carotid artery) or central cannulation (drained from right atrium and perfused to ascending aorta) via median sternotomy. There are disadvantages with both these approaches. While neck cannulation has a greater risk of neurologic injury, central cannulation has greater risks of mediastinitis and bleeding [34].
The cannulation strategy, therefore, is made as a balance of the risks/benefits of the chosen vessel and the desired blood flow. As a rule of thumb, the desired blood flow is that which perfuses all end organs adequately. Although most units might start at about 120–150 mL/kg/min in a neonate (cardiac index 2.2–2.4 L/m2/min in an older child), this will then be titrated up or down according to the requirements of the child. Ideally, markers of end-organ perfusion such as arterial lactate, mixed venous saturations, preoxygenator arterial oxygen saturations, urine output, biochemical variables of liver and renal function, and mean arterial blood pressure (5th centile for the age) are monitored closely to optimise ECMO support [34].
3. Left heart decompression
Left heart decompression (LHD) facilitates myocardial recovery in ECMO patients. ECMO increases the afterload of the left heart and this can lead to left atrial and left ventricular (LV) dilation resulting in pulmonary oedema and thrombus formation within the cardiac chambers [35]. Additionally, the volume overload increases myocardial wall stress and induces inflammatory reactions. Consequently, in inflammatory diseases such as AFM, the combination of LV overloading and inflammation may lead to unfavourable cardiac remodelling. By unloading the LV, wall stress and inflammatory responses are reduced [36].
As LHD is needed in 13% to 46% of pediatric VA-ECMO cases [37-39], certain warning signs might enable decision making. In a normally septated heart with no native ejection (a continuously flat arterial line waveform), ECG trace of ventricular tachycardia, ventricular fibrillation, pulseless electric activity, agonal heart rhythm, or asystole could be critical signals. A persistently closed aortic valve, LV dilatation, sludge, or thrombus formation in LV revealed by echocardiography are additional pointers [39].
LHD can be achieved by percutaneous techniques or surgery. It can be performed by atrial septostomy or by placing a venting catheter in the left atrium or left ventricle. Although LHD demonstrated no survival benefits in some studies [38,40], a recent meta-analysis of adult cardiogenic shock reported an association with decreased mortality. LHD was also associated with a higher rate of LV recovery, relief of pulmonary oedema, weaning off, and duration of ECMO [38,41,42]. Studies that investigated LHD are summarised in Table 3.
4. Anticoagulation, bleeding, and thrombosis
ECMO creates interactions between blood and the biomaterial interface, which results in activation of inflammatory and coagulation cascades [43,44]. Thus, anticoagulation becomes mandatory. Optimal anticoagulation is required to limit thrombotic and bleeding complications. Achieving this ‘optimal’ goal has been challenging.
The optimal management of the coagulation system in children is unclear [45-47]. Developmental haemostasis precludes extrapolation of adult anticoagulation strategies. Unfractionated Heparin (UFH) remains the standard of care in pediatric ECMO. However, reports of age-dependent variation in UFH activity with paucity of pediatric research in this area yield varying practices between institutions [48-50]. Research to find a better alternative is underway. Direct thrombin inhibitors (e.g., bivaluridin) have shown promise in early studies [51,52]. Although there are no standard methods for monitoring the effects of anticoagulants in neonatal and pediatric patients on ECMO, the 3 common assays are commonly used which are activated clotting time, activated partial thromboplastin time, and Anti-Xa [53-55]. Table 3 summarises studies that investigate anticoagulation practices in paediatric ECMO.
Thromboembolic and hemorrhagic complications are a frequent cause of death in children supported on ECMO. According to the ELSO registry, the most common mechanical complications are clots in circuits, complicating 22% of neonatal and 17% of pediatric cardiac ECMO runs [2]. Unfortunately, neurologic complications (secondary to bleeding or emboli) are more frequently observed in children than adults [56]. Of note, intracranial haemorrhage, cerebral infarction, and seizures are all seen twice as often in children compared to adults [57]. In addition, regardless of age, neck cannulation increases the risk of neurologic injury in children. The derangement of cerebral autoregulation, the decrease in cerebral venous drainage, and an increased risk of thromboembolic events are possible hazards of this approach [58]. Due to these potentially significant complications, patients on ECMO are closely monitored with regular anticoagulation assays. In the near future, routine neuromonitoring strategy inclusive of techniques such as near infrared spectroscopy and electro-encephalograms might become standard of care [59]. Table 4 summarises studies that explored bleeding, thrombosis, and neurologic complications during pediatric ECMO.
5. Weaning from ECMO
When cardiac function has recovered adequately, the patient is assessed for separation from ECMO support. The main variables that dictate successful weaning are echocardiographic evidence of myocardial recovery, with a good lung function (adequate gas exchange on less than moderate ventilatory support), resolution of end-organ dysfunction, and optimal pulse pressure/end tidal CO2 [34,62]. Echocardiographic evaluation should ideally be made under low-flow conditions. The specific parameters shown to predict are aortic velocity time integral >10 cm, LV EF >20%–25%, and lateral mitral annulus peak systolic velocity ≥6 cm/sec [63].
6. Escalation of therapy from ECMO
In a small proportion of AFM patients on ECMO, cardiac function might not adequately recover within 2 weeks. In these instances, ventricular assist devices (VADs) or listing for a heart transplantation maybe considered [14,16,28,37,64,65]. While discussion with parents and preparation should start early, the diagnosis and decision need carful thought. Following prerequisites lend themselves a guide for the decision making around transition to VAD or heart transplantation [66,67]:
•Potential recovery of heart function has been quantified
•End-organ dysfunction has been shown to be reversible
•Lung function is adequate
•Right ventricle is expected to cope with no support (in case of left VAD placement)
•Neurological function has been quantified to best of ability
•Recovery of cardiac function is expected to take more a few weeks (in BTR strategy)
•Patient has been listed for heart transplant (BTT strategy) and age/weight/blood group considerations might delay potential for organ availability
Although survival rate in transplant patients is also favourable [14,28,37,64], the role of heart transplantation has been questioned, as it has become more apparent that native ventricular function is ultimately expected to return, and prolonged duration of extracorporeal support can salvage these patients to recovery [64,68].
7. Outcomes
Even though AFM often presents as cardiogenic shock or cardiac arrest, the long-term survival is favourable [69]. Similarly, patients with AFM on ECMO have relatively good survival rates ranging from 58% in neonates to 63%–81% in pediatric patients [2,30,58,70]. The parameters associated with mortality are female gender, arrhythmia during ECMO support, renal failure requiring dialysis, and higher stage of end-organ damage [40,64,71].
Similar to neuromonitoring, the long-term neurological outcome in pediatric AFM patients supported on ECMO has not been reported. But some of this can be extrapolated from reports on children with other conditions who survived with ECMO. Alarmingly, lower intelligence quotient scores, behaviour problems, and severe neurodevelopmental delays have been reported in post ECMO pediatric survivors [69,72]. These findings warrant long-term follow-up not only to monitor recovery of cardiac function but also to delineate neurological functional deficits.
1) Timing of ECMO initiation and outcome
The decision to place a patient on ECMO is not easy. The clinician has to balance the risk and the benefit of such an invasive intervention. Clear guidelines cannot be stipulated for this scenario as each patient, each clinical setting, and each team bring in a myriad of nuances. With a haemodynamic profile similar to the AFM patient, data from patients in septic shock requiring ECMO could provide some insights. Schlapbach et al. [73] created a predicted mortality risk for septic controls. This was then compared to the observed mortality for those who were placed on ECMO. They derived a treatment benefit threshold of 47.1% predicted risk of mortality. Above this cutoff, patients were better off on ECMO, while below this value patients did well without ECMO. These results, if extrapolated to other groups, suggest that too early application of ECMO leads to higher mortality due to complications of the therapy, while too late application leads to higher mortality due progression of the underlying disease with inherent complications [73,74].
Conclusions
The early recognition of the clinical signs of a failing heart is paramount. Outcomes in children with AFM are favourable when ECMO is instituted before end-organ dysfunction ensues. Hence, early discussion and implementation of ECMO are mandatory to avoid potentially lifelong sequelae through distant organ injury. The clinician must be cognisant of the complications related to ECMO. This should guide decision making and parental counselling. Finally, a multidisciplinary team approach along with parents is needed to achieve better survival and quality of life. Key uncertainties that clinicians face when managing this patient cohort are the timing of initiation of ECMO and LV decompression. These need to be urgently investigated with strong research methodologies.
Notes
No potential conflict of interest relevant to this article was reported.
Acknowledgements
Hwa Jin Cho acknowledges the support of a grant NRF-2018R1D1A1A02085975 from National Research Foundation of Korea and a grant BCRI18028 from the Chonnam National University Hospital Biomedical Research Institute. Silver Heinsar received a PhD Scholarship from The Prince Charles Hospital Foundation.
Authors acknowledge Sarfaraz Rahiman and In Seok Jeong for reviewing the paper before the submission and Dr. Samia Farah, MD for the illustration, which was created with BioRender.com.