Expression of peroxisome proliferator-activated receptor (PPAR)-α and PPAR-γ in the lung tissue of obese mice and the effect of rosiglitazone on proinflammatory cytokine expressions in the lung tissue
Article information
Abstract
Purpose
We investigated the mRNA levels of peroxisome proliferator-activated receptor (PPAR)-α, PPAR-γ, adipokines, and cytokines in the lung tissue of lean and obese mice with and without ovalbumin (OVA) challenge, and the effect of rosiglitazone, a PPAR-γ agonist.
Methods
We developed 6 mice models: OVA-challenged lean mice with and without rosiglitazone; obese mice with and without rosiglitazone; and OVA-challenged obese mice with and without rosiglitazone. We performed real-time polymerase chain reaction for leptin, leptin receptor, adiponectin, vascular endothelial growth factor (VEGF), tumor necrosis factor (TNF)-α, transforming growth factor (TGF)-β, PPAR-α and PPAR-γ from the lung tissue and determined the cell counts and cytokine levels in the bronchoalveolar lavage fluid.
Results
Mice with OVA challenge showed airway hyperresponsiveness. The lung mRNA levels of PPARα and PPAR-γ increased significantly in obese mice with OVA challenge compared to that in other types of mice and decreased after rosiglitazone administeration. Leptin and leptin receptor expression increased in obese mice with and without OVA challenge and decreased following rosiglitazone treatment. Adiponectin mRNA level increased in lean mice with OVA challenge. Lung VEGF, TNF-α, and TGF-β mRNA levels increased in obese mice with and without OVA challenge compared to that in the control mice. However, rosiglitazone reduced only TGF-β expression in obese mice, and even augmented VEGF expression in all types of mice. Rosiglitazone treatment did not reduce airway responsiveness, but increased neutrophils and macrophages in the bronchoalveolar lavage fluid.
Conclusion
PPAR-α and PPAR-γ expressions were upregulated in the lung tissue of OVA-challenged obese mice however, rosiglitazone treatment did not downregulate airway inflammation in these mice.
Introduction
Epidemiological data show an increased incidence of asthma in overweight or obese children and adults. The relative risk of incident asthma increases with increasing body mass, which has been associated with enhancement of asthma symptoms, airway hyperresponsiveness (AHR) and atopy1). Obesity is particularly important for severe asthma because more than 75% of asthmatic individuals visiting the emergency room are obese or overweight1). Obesity may be related to the steroid-resistant asthma.
Consumption of a high-fat diet has been shown to lead to lipid accumulation and inflammatory signaling in key neuronal subsets involved in the regulation of biochemical resistance to insulin, leptin, and other regulatory hormones and nutrient signals2).
In order to understand the effect of dietary fat on obesity predisposition, we sought to identify molecular metabolic regulators that may be lipid sensitive. Peroxisome proliferator-activated receptor (PPAR)-γ is member of the PPAR family of nuclear receptors, a class of lipid activated transcription factors belonging to the nuclear receptor superfamily2).
Current evidence suggests that obesity related changes in the production of adipose-derived cytokines, adipokines, may modulate asthma. Alterations in the production of the adipokines such asleptin and adiponectin could play a critical role in mediating airway inflammation and AHR3).
PPARs are a family of ligand-activated transcription factors belonging to the nuclear hormone receptor family and related to retinoid, glucocorticoid, and thyroid hormone receptors4). The presence of PPARs has been demonstrated in a variety of cells associated with lung inflammation5). The widespread distribution of PPAR in the airway suggests that they may play important roles in both lung health and disease6).
PPARs can be involved in the inflammatory cascade of asthma, and treatment with PPAR agonists may reduce airway inflammation4). The activation of PPAR-γ and PPAR-α is thought to down-regulate proinflammatory gene expression and inflammatory cell functions4). PPAR-γ ligands inhibit the release of proinflammatory cytokines from activated macrophages, airway epithelial cells, and eosinophils, and decrease AHR, basement membrane thickness, collagen deposition, and eosinophilia in murine models of asthma7).
There have been a few studies for the effect of PPAR-γ agonist on airway inflammation in asthmatics, however there is no data on obesity, especially on obese asthma. The presents study aimed to compare the mRNA levels of lung PPAR-γ and PPAR-α among lean and obese mice with and without ovalbumin (OVA) challenge and investigate the effect of rosiglitazone on cytokines related to airway inflammation and airway responsiveness.
Materials and methods
We developed a mouse model of chronic asthma with or without obesity. Mice were divided into 7 groups: control group, lean OVA-challenged groups with (A) and without (B) rosiglitazone, obese groups with (C) and without (D) rosiglitazone, and obese OVA-challenged groups with (E) and without (F) rosiglitazone. Each group was comprised of 6 mice. The animal experimental protocol was approved by the Animal Subjects Committee.
1. Sensitization and antigen challenge protocol
1) Obese model
Six-week-old female C57BL/6J mice (Central Lab. Animal Inc., Seoul, Korea) were fed a high-fat diet in which 45% of the calories are derived from fat (Feedlab, Guri, Korea) for a period of 16 weeks. Food intake and body weight were measured every 7 days during the study period. Mice were allowed free access to water and food and housed 4 per cage in a temperature-controlled room with a 12:12-hour light-dark cycle. Nonobese mice were given a standard diet. Rosiglitazone was administered with diet in 0.01% concentration.
2) Chronic asthma model
Six-week-old female C57BL/6J mice were immunized by subcutaneous injection on days 0, 7, 14, and 21 with 25 µg of OVA (grade V; Sigma-Aldrich Co., St. Louis, MO, USA) adsorbed to 1 mg of aluminum hydroxide (Sigma-Aldrich Co.) in 200 µL of phosphate-buffered saline (PBS). Intranasal OVA challenges (20 µg/50 µL in PBS) were administered on days 27, 29, and 31 under isoflurane anesthesia. Intranasal OVA challenges were repeated twice per week until week 18 of the study period. Age- and sex-matched control mice were treated the same way with PBS but without OVA. Mice were sacrificed 24 hours after the final OVA challenge, and bronchoalveolar lavage (BAL) and lung tissues were obtained.
3) Measurement of AHR
AHR was assessed by a single-chamber whole-body plethysmograph (Allmedicus, Anyang, Korea). The enhanced pause (Penh) was used to monitor airway responsiveness. In the plethysmograph, mice were exposed for 3 minutes to nebulized PBS to establish baseline Penh values, and were subsequently exposed to increasing concentrations of nebulized methacholine (3.50 mg/mL; Sigma-Aldrich Co.) in PBS using an Aerosonic ultrasonic nebulizer (DeVilbiss, Somerset, PA, USA). Following each nebulization, recordings were taken for 3 minutes. The Penh values measured during each 3-minute sequence were averaged and are expressed for each methacholine concentration.
4) Analysis of cells in BAL fluid
Mice were sacrificed after measuring AHR. The trachea was exposed and cannulated with silicone tubing attached to a 23-gauge needle on a 1 mL syringe. After the instillation of 1 mL of sterile PBS into the lung, the BAL fluid was withdrawn. The BAL fluid was stained with a hematology analyzer, XE-2100 (Sysmex, Kobe, Japan) after cytospinning (7 minutes at 2,000 rpm). Total cell count was performed using a hemacytometer. After counting 400 leukocytes on the slide by light microscopy, the percentage of eosinophils, neutrophils, lymphocytes, and macrophages were calculated. Supernatants were stored at -70℃.
5) Analysis of vascular endothelial growth factor (VEGF) and transforming growth factor (TGF)-β in BAL fluid
VEGF and TGF-β concentrations were measured using enzyme-linked immunosorbent assay kit (R&D, Minneapolis, MN, USA) in BAL fluid according to the manufacturer's instructions.
6) RNA isolation and real-time reverse transcription-polymerase chain reaction (RT-PCR)
Lung tissues were analyzed by real-time PCR for leptin, leptin receptor, adiponectin, adiponectin receptor (Adipor1 & Adipor2), VEGF, TGF-β, and TNF-α. Total RNA was isolated from lung tissue using Trizol reagent (Invitrogen, Carlsbad, CA, USA). Total RNA, at a concentration of 2 µg, was reverse-transcribed with Moloney murine leukemia virus reverse transcriptase (MMLV-RT) and oligo8) 12-18 primer (Invitrogen), to generate cDNA. Briefly, RNA was denatured for 10 minutes at 72℃, then immediately placed on ice for 5 minutes. Denatured RNA was mixed with MMLV-RT, MMLV-RT buffer, and dNTP mixture, and incubated for 1 hour at 42℃. The reagent was inactivated by heating at 95℃ for 2 minutes. Synthesized cDNA was amplified by real-time PCR (Light-Cycler 480, Roche, Lewis, UK) using SYBR green (Invitrogen) and specific primers (Bioneer Co., Daejon, Korea). The following primers were used: leptin, forward: 5'-TGGCTTTGGTCCTATCTGTC-3', reverse: 5'-TCCTGGTGACAATGGTCTTG-3' (GenBank accession number: BC125245); leptin receptor, forward: 5'-TGTCTCAGCTACATCTCTGC-3', reverse: 5'-CTGAACCATCCAGTCTCTTG-3' (GenBank accession number: BC082551); adiponectin, forward: 5'-AGAGAAAGGAGATGCAGGTC-3', reverse: 5'-TGAACGCT GAGCGATACACA-3' (GenBank accession number: NM009605); Adipor1, forward: 5'-AGATGGGCTGGTTCTT CCTC-3', reverse: 5'-CAGACAACTCAGACTCTTCC-3' (GenBank accession number: NM1283203); Adipor2, forward: 5'-ATGTTTGCCACCCCTCAGTA-3', reverse: 5'-CAGATGTCACATTTGCCAGG-3' (GenBank accession number: NM197985); VEGF, forward: 5'-GTGGACATCTTCCAGGAGTA-3', reverse: 5'-TTCATCGTTACAGCAGCCTG-3' (GenBank accession number: BC061468); TGF-, forward: 5'-AGTGTGGAGCAACATGTGGA-3', reverse: 5'-GTACAACTCCAGTGACGTCA-3' (GenBank accession number: BC013738); TNF-α, : 5'-CGCTCTTCTGTCTACTGAAC-3', reverse: 5'-CTACAGGCTTGTCACTCGAA-3' (GenBank accession number: NM013693). Each cycle consisted of denaturation at 94℃ for 15 seconds, annealing at 55℃ for 10 seconds, and extension at 72℃ for 20 seconds. Quantification was performed using the comparative 2-(delta delta Ct) method; expression levels for the target genes were normalized to the β-actin of each sample.
2. Data analysis
Results for each group were compared by analysis of variance, followed by Dunn's multiple comparison of means. SPSS ver. 10.0 (SPSS Inc., Chicago, IL, USA) was used for analysis. A P value of <0.05 was considered statistically significant. All results are given as mean±standard error of the mean.
Results
1. Effect of high-fat diet on body weight
Obese groups fed 45% high-fat diet with and without OVA challenge weighed significantly more than other groups fed a normal diet. At the end of the study, mice in group C and D reached 27.8 g and 27.7 g, while control mice weighted 22.5 g, and 23% (9.6 g weight gain) and 24% (9.9 g weight gain) more weight gain than that of control group (4.5 g weight gain), respectively (P<0.05). Groups E and F gained more weight by 16% (8.3 g weight gain) and 14% (7.7 g weight gain) compared to control group, respectively (P<0.05). Groups A and B miceshowedno difference in weight (4.5 g and 2.7 g weight gain, respectively) compared to control mice (Fig. 1).
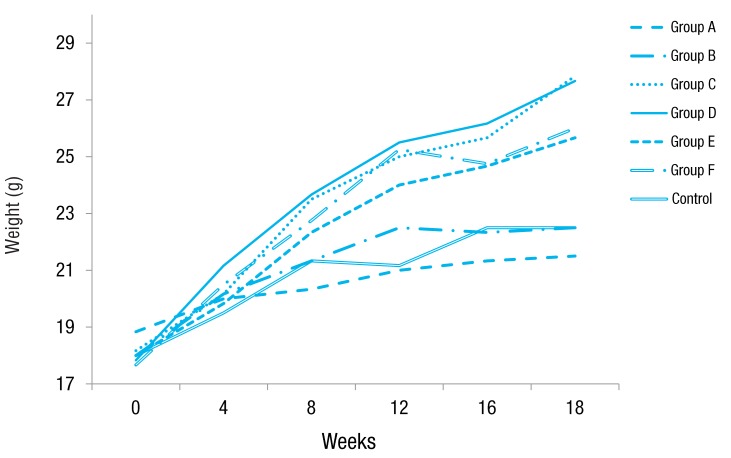
Effect of high-fat diet on body mass. Mice fed a high-fat diet (groups C, D, E, and F) weighed significantly more than control mice, whereas mice fed a normal diet (groups A and B) showed no difference in weight compared to control mice at after 18 weeks of the study period. Group A, lean mice with ovalbumin (OVA) challenge; group B, rosiglitazone-treated lean mice with OVA challenge; group C, obese mice without OVA challenge; group D, rosiglitazone-treated obese mice without OVA challenge; group E, obese mice with OVA challenge; and group F, rosiglitazone-treated obese mice with OVA challenge.
2. Airway inflammation
Total cell numbers and macrophage numbers in BAL fluid increased significantly in groups A to F mice compared to the control group (P<0.05). Groups A, B, E, and F mice also showed a significant increase in total cell and macrophage numbers compared to groups C and D mice (P<0.05). Groups A, B, E and F mice had significantly increased number of eosinophils in the BAL fluid compared to those in the control group (P<0.05), whereas groups C and D mice showed no significant increase in eosinophils compared to control group. Mice in groups C, D, E, and F showed significant increases in neutrophil numbers in BAL fluid compared to control mice (P<0.05). Rosiglitazone treatment didn't affect cell numbers in BAL fluid compared to no-treatment in each group (Fig. 2).

Effect of obesity and ovalbumin (OVA) challenge on total and differential cell counts in the bronchoalveolar lavage (BAL) fluid. The mice in groups A, B, E, and F showed a significant increase in the numbers of total cells, eosinophils, and macrophages compared to control mice and mice in groups C and D. Compared to the control group, the mice in groups C and D showed increased numbers in total cell and macrophage counts, but not in the number of eosinophils. The number of neutrophils increased significantly in the mice in groups C, D, E, and F compared to that in control mice. group A, lean mice with OVA challenge; group B, rosiglitazone-treated lean mice with OVA challenge; group C, obese mice without OVA challenge; group D, rosiglitazone-treated obese mice without OVA challenge; group E, obese mice with OVA challenge; and group F, rosiglitazone-treated obese mice with OVA challenge. *P<0.05 vs. control, groups C, and D mice. †P<0.05 vs. control mice.
3. AHR to methacholine
Increasing doses of methacholine induced an increase in airway resistance in all types of mice. The Penh increase was higher in groups A, B, E and F mice than in control mice, revealing the induction of AHR in OVA-challenged mice. However, groups C and D mice didn't show AHR. Rosiglitazone treatment did not affect AHR in each type of mice with no rosiglitazone treatment (Fig. 3).

Airway reactivity towards increasing concentrations of methacholine. Mice in groups A and B showed airway reactivity at methacholine dose of 12.5 mg/mL, group E mice at 25 mg/mL, and group F mice at 50 mg/mL compared to control mice. However, mice in groups C and D did not show airway hyperresponsiveness compared to control mice. group A, lean mice with ovalbumin (OVA) challenge; group B, rosiglitazone-treated lean mice with OVA challenge; group C, obese mice without OVA challenge; group D, rosiglitazone-treated obese mice without OVA challenge; group E, obese mice with OVA challenge; and group F, rosiglitazone-treated obese mice with OVA challenge. *P<0.05 vs. control.
4. Expression of PPAR-α, PPAR-γ, leptin, leptin receptor and adiponectin mRNA in lung tissue of mice
To assess the effect of obesity on lung inflammation and expression of adipokines in mice, we measured mRNA levels of PPAR-α, PPAR-γ, leptin, leptin receptor, adiponectin, adiponectin receptor, VEGF, TNF-α and TGF-β in lung tissue of mice by real-time PCR.
Expression of PPAR-α and PPAR-γ increased significantly in group E mice compared to control mice, which decreased significantly when rosiglitazone was treated (group F). There were no differences in PPAR-α and PPAR-γ expressions in groups A, B, C, and D mice compared to control mice (Fig. 4A).

ThemRNA levels of peroxisome proliferator-activated receptor (PPAR)-γ, PPAR-α, leptin, leptin receptor, and adiponectinin the lung tissue of mice and the effect of rosiglitazone treatment. Expression of PPAR-α and PPAR-γ increased significantly in group E mice compared to that in control mice, and it decreased significantly with rosiglitazone treatment in group F mice (A). Leptin and leptin receptor increased significantly in the mice in groups C and E compared to that in control mice, and these decreased significantly with rosiglitazone treatment. Adiponectin increased significantly in group A mice compared to that in control mice, and rosiglitazone augmented adiponectin mRNA level in group D mice compared to that in group C mice (B). Group A, lean mice with ovalbumin (OVA) challenge; Group B, rosiglitazone-treated lean mice with OVA challenge; Group C, obese mice without OVA challenge; Group D, rosiglitazone-treated obese mice without OVA challenge; Group E, obese mice with OVA challenge; and Group F, rosiglitazone-treated obese mice with OVA challenge. *P<0.05 vs. control. †P<0.05 vs. each group of mice without rosiglitazone treatment.
Leptin and leptin receptor mRNA levels increased in groups C and E mice compared to control mice, and rosiglitazone decreased leptin and leptin receptor mRNA levels in both mice (groups D and F). However, administration of rosiglitazone showed increased expression of leptin and leptin receptor mRNA levels in group B mice compared to group A mice.
Adiponectin mRNA levels increased significantly in group A mice compared to control mice and showed a tendency of decrease in group C mice. Administration of rosiglitazone increased adiponectin mRNA levels in group D mice compared to group C mice, while it showed decreased tendency in group B mice compared to group A mice (Fig. 4B).
5. VEGF, TNF-α, and TGF-β levels in BAL fluid and mRNA expression in lung tissue of mice
VEGF mRNA levels increased significantly in groups A, C, and E mice compared to control mice, which augmented more when rosiglitazone was administered (groups B, D, and F, respectively) compared to no rosiglitazone treatment. Lung mRNA levels of TNF-α and TGF-β increased significantly in groups C and E mice compared to control mice. Rosiglitazone also increased TNF-α mRNA levels in group F mice compared to group E mice, however decreased TGF-β mRNA levels in group D mice compared to group C mice (P<0.05) (Fig. 5A).

The mRNA levels of vascular endothelial growth factor (VEGF), transforming growth factor (TGF)-β, and tumor necrosis factor (TNF)-α in the lung tissue of mice and the effect of rosiglitazone in the bronchoalveolar lavage (BAL) fluid. VEGF expression in the lung increased significantly in the mice in groups A, C, and E and was augmented significantly after rosiglitazone treatment. TNF-α and TGF-β expression in the mice in groups C and E increased significantly compared to that in control mice. TNF-α expression was augmented with rosiglitazone treatment in group F mice compared to that in group E mice, while TGF-β expression decreased significantly in group D mice compared to that in group C mice (A). VEGF concentration in the BAL fluid was higher in the mice in groups A, C, and E than in control mice and increased significantly in each group of mice with rosiglitazone treatment (groups B, D, and F). TGF-β level in the BAL fluid was higher in the mice groups C and E than in control mice and decreased significantly in group D mice with rosiglitazone treatment (B and C). Group A, lean mice with ovalbumin (OVA) challenge; group B, rosiglitazone-treated lean mice with OVA challenge; group C, obese mice without OVA challenge; group D, rosiglitazone-treated obese mice without OVA challenge; group E, obese mice with OVA challenge; and group F, rosiglitazone-treated obese mice with OVA challenge. *P<0.05 vs. control. †P<0.05 vs. each group of mice without rosiglitazone treatment.
VEGF levels in BAL fluid were also higher in groups A, C, and E mice than in control mice, which increased significantly in rosiglitazone-treated mice (groups B, D, and F, respectively). Concentration of TGF-β in BAL fluid augmented in groups C and E mice compared to control mice, which decreased significantly in group D mice compared to group C mice (Fig. 5B, C).
Discussion
We compared PPAR-γ and PPAR-α mRNA levels in lung tissue of mice with OVA challenge and/or obesity, and also investigated the effect of rosiglitazone on lung expressions of PPAR-γ and PPAR-α. PPAR-γ and PPAR-α mRNA levels increased significantly in obese mice with OVA challenge compared to lean mice with OVA challenge and obese mice as well as control mice, and decreased significantly when rosiglitazone was treated.
PPAR-γ has been suggested as an indicator of airway inflammation and remodeling in asthma9). A study indicated a positive correlation between PPAR-γ expression and airway remodeling in asthma such as subbasement membrane thickening, collagen deposition, and increase of proliferating cells in the epithelium and submucosa area10). Intensity of PPAR-γ expression in the bronchial submucosa, the airway epithelium and the smooth muscle also showed negative correlation with the forced expiratory volume values. Furthermore, steroids restrained PPAR-γ expression in lung tissue10). Expression of PPAR-γ is also related to obesity. In our previous study, PPAR-γ expression also increased in obese rat11). We found PPAR-γ mRNA level was higher in obese mice with OVA challenge than obese mice or lean mice with OVA challenge, which suggests synergistic effect of obesity and asthma on PPAR-γ expression. To our knowledge, there has been no report that investigated PPAR-γ and PPAR-α expressions in obese mice with OVA challenge.
Asthma is a chronic inflammatory airway disease, and obesity also represents a low-grade chronic inflammatory state. Considering obese patients have more difficulties in controlling asthma and decreased responsiveness to inhaled corticosteroid12), PPAR-γ or PPAR-α agonist can be a good candidate for controlling difficult asthma in obese asthmatics.
There are a few clinical studies for the therapeutic effect of PPAR-γ ligands in asthma. Pioglitazone improved wheezing and coughing in patients with asthma, and asthma symptoms restarted when the pioglitazone treatment was discontinued13). Another study shows that rosiglitazone improved lung function, compared with inhaled corticosteroid in steroid-resistant smoker with asthma14), and PPAR-γ agonists reduced the asthma symptoms and levels of Th2 cytokines, adhesion molecules, chemokines, and TGF-β1 in toluene diisocyanate-induced asthma15). On the contrary, PPAR-γ genetic expression has been shown to be reduced in asthmatic patients after an allergen challenge, and inversely related to airway inflammation16). In mature adipocytes, PPAR-γ interacts with several coactivators with chromatin modifying activities, repress and enhance its transcriptional activity17). Although PPAR-γ and PPAR-α lung expressions didn't increase significantly in mice with OVA challenge and with obesity, we found a tendency of increase. As a result, rosiglitazone administration decreased the lung PPAR-γ and PPAR-α mRNA levels in mice with OVA challenge. We can figure out the possibility of significance with the increase of study subjects in the future study.
The direct mechanism for the relationship between obesity and asthma has not been established yet. Leptin is a hormone secreted preferentially by adipose tissue, exerting pleiotropic effects such as body weight homeostasis, reproduction and hematopoiesis18). Leptin acts as a proinflammatory cytokine, and its blood level is elevated in obese people. Leptin receptor is expressed in airway smooth muscle19), and leptin promoted VEGF release by human airway smooth muscle cells20). In this study, leptin as well as leptin receptor demonstrated increased mRNA levels in obese mice with and without OVA challenge, which decreased with rosiglitazone. However, they didn't show significant increase in mice with OVA challenge compared to control mice, and there was no difference in the expression of leptin and leptin receptor between obese mice with and without OVA challenge. Rosiglitazone administration to the preadipocytes isolated from obese mice, resulted in the differentiation into mature adipocytes. The inhibitory effect of leptin on rosiglitazone induced adipocyte differentiation was also accompanied by the decreased expression of adipogenic transcription factors. Rosiglitazone was decreased in the presence of leptin18). Leptin may be related to obesity itself, not asthma, and may not be a contributing factor for poorly controlled asthma in obese asthmatics. There has been no data onthe effect of rosiglitazone on leptin regulation in asthma. Contrary to our study, another study demonstrated rosiglitazone increased leptin levels in obese mice, while decreased leptin levels in lean mice3). However, they tried short-course 1 week treatment of rosiglitazone on airway as opposed to 18 weeks administration in this study.
Adiponectin may play an anti-inflammatory role in obesity related diseases21). Adiponectin receptors are expressed in airway smooth muscle20). In this study, lung expression of adiponectin increased significantly in mice with OVA challenge, and decreased in obese mice. However, there was no significant difference in obese mice with OVA challenge compared to control mice. Until now, there has been no data which investigated the lung expression of adiponectin in obese mice with OVA challenge. Lung adiponectin may not be a major factor to regulate the airway inflammation in obese asthmatics.
Proinflammatory cytokines such as TNF, TGF, and VEGF are increased in obesity. They are also an important cytokines in regulating airway inflammation in asthma20,22,23). We found augmented lung expression of VEGF, TNF-α, and TGF-β in both obese mice with and without OVA challenge, and VEGF mRNA level also increased in lean mice with OVA challenge. However, there was no synergistic increase of cytokine expressions in obese mice with OVA challenge compared to lean mice with OVA challenge or obese mice. In this study, rosiglitazone enhanced lung VEGF mRNA level in all types of mice, and TNF-α mRNA level in obese mice with OVA challenge. This is supported by another study with murine model with chronic asthma, which shows increased VEGF and TGF-β levels in BAL fluid of ciglitazone-treated asthma mice24). However, other studies showed PPAR agonists decreased serum VEGF levels and lung expression of TGF-β7,25), and reduced expression of TNF-α in adipocytes ofobese mice treated with the PPAR-γ agonist troglitazone26). We can assume VEGF, TNF-α, and TGF-β are not major contributing cytokines regulated by rosiglitazone in obese asthmatics. There has been no data on the effect of rosiglitazone on cytokine expressions in obese asthmatics.
This study was limited by relatively small number of subjects and no measurement of AHR and airway inflammation. However, this is the same method to develop chronic asthma model in mice24). And we administered rosiglitazone into the diet, not into the airway. The method and type of PPAR-γ agonist as well as the duration of administration can make difference. However, there has been only a few studies using murine model of obese asthmatics, and to our knowledge, this is the first study comparing lung expression of PPAR, adipokines, and cytokines according to obesity and OVA challenge.
Taken together, this study suggests that PPAR-α and PPAR-γ lung expression is augmented in obese mice with OVA challenge, and PPAR-γ agonist dose not down-regulate VEGF, TNF-α, and TGF-β expressions in lung tissue of obese asthma.
Acknowledgments
This study was supported by Kangbuk Samsung Hospital Medical Research Fund.
Notes
No potential conflict of interest relevant to this article was reported.