All issues > Volume 66(3); 2023
Ferritin in pediatric critical illness: a scoping review
- Corresponding author: Dyah Kanya Wati. Department of Child Health, Faculty of Medicine, Universitas Udayana/Sanglah General Hospital, Diponegoro Street, Dauh Puri Klod 80113 – Denpasar, Indonesia Email: dyahkanyawati@unud.ac.id
- Received May 6, 2022 Revised July 27, 2022 Accepted August 17, 2022
- Abstract
-
This scoping review aimed to elucidate and summarize the predictive role of serum ferritin in critical pediatric illness. The Preferred Reporting Items for Systematic reviews and Meta-Analyses methodology was employed to conduct a scoping review of 5 databases (MEDLINE, CENTRAL, ProQuest, ScienceDirect, and Epistemonikos) from the date of inception through January 24, 2022. Primary research studies involving subjects aged <18 years and serum ferritin levels were screened and reviewed independently following an a priori defined protocol. Of the 1,580 retrieved studies, 66 were analyzed. Summary statistics of serum ferritin levels for overall and condition-specific studies were reported in 30 (45.4%) and 47 studies (71.2%), respectively. The normal range was defined in 16 studies (24.2%), whereas the threshold was determined in 43 studies (65.1%). A value of <500 ng/mL was most often the upper limit of the normal range. Serum ferritin as a numerical variable (78.9%) was usually significantly higher (80.8%) in the predicted condition than in controls, while as a categorical variable with preset thresholds, ferritin was a significant predictor in 84.6% of studies. A total of 22 predictive thresholds predicted mortality (12 of 46 [26.1%]), morbidity (18 of 46 [39.1%]), and specific (16 of 46 [34.8%]) outcomes in 15 unique conditions. Increased precision in serum ferritin measures followed by close attention to the threshold modeling strategy and reporting can accelerate the translation from evidence to clinical practice.
- Introduction
- Introduction
Ferritin is a spherical protein composed of a protein shell that encapsulates an iron core. The shell consists of 24 protein subunits called apoferritin, with a certain ratio of H and L chains according to tissue type. The Fe3+ state of iron is stored in the iron core [1]. This iron storage protein can be found in virtually all cells with cytosolic, mitochondrial, and nuclear localization. Since its discovery in 1937 and quantification in human serum in 1972, the role of ferritin in clinical practice has expanded beyond its well-established function in iron homeostasis [2]. Areas of ferritin utility in diagnostic and prognostic studies have evolved considerably from indicators of iron storage, acutephase reactants, disease biomarkers, and recently discovered hyperferritinemic syndrome [3].Despite the decreasing trend in the global pediatric mortality rate in the pre-coronavirus disease 2019 (COVID-19) pandemic period, the morbidity rate continued to increase owing to unresolved issues caused by acute illnesses [4]. Pre- and pandemic comparisons of pediatric critical illness trends revealed increasing mortality rates despite concurrent lower critical care admission rates [5,6]. Considering that both acute potentially fatal illness and medical complexity resulting from morbidity are subject to critical care management [7], this evidence suggests the need for evidence-based critical care improvements, especially those that enable the early prediction of critical illness outcomes to prevent mortality and morbidity [8,9].Ferritin is among the most thoroughly investigated biomarkers for predicting outcomes under various conditions [10-13]. It was also included as a defining criterion for the critical COVID-19 spectrum, specifically in the pediatric population, namely multisystem inflammatory syndrome in children (MIS-C) and pediatric multisystem inflammatory syndrome temporally associated with COVID-19 [14,15]. However, a systematic review of their multifaceted predictive ability is lacking. This systematic scoping review aimed to: (1) identify predictive serum ferritin threshold(s); (2) investigate targeted clinical conditions; and (3) map available outcomes.
- Methods
- Methods
- 1. Sources and search strategy
- 1. Sources and search strategy
Searches were conducted in MEDLINE (PubMed), CENTRAL (Cochrane Library), ProQuest, ScienceDirect, and Epistemonikos using combined keywords and controlled vocabulary (pediatric, critical illness, and ferritin) and their synonyms connected with appropriate Boolean operators (AND and OR). The search strategy was initially constructed for PubMed before it was adapted to the other databases (Supplementary Material 1). An additional search for gray literature was performed to locate unpublished studies. Filters for articles written in English and full-text availability were applied where possible, and all search results from the date of inception to January 24, 2022, were included.- 2. Study selection
- 2. Study selection
The inclusion criteria were as follows: (1) primary research study; (2) subjects aged <18 years; and (3) serum ferritin measurement results. The exclusion criteria were as follows: (1) secondary research study; (2) nonresearch publication type; (3) noncritical illness or condition; (4) pediatric outcomes not separated from adult outcomes; and (5) ferritin measurement not presented.The search results from all databases were downloaded as reference lists and combined to check for duplicates using reference management software. After duplicate removal, the combined references were managed using the Rayyan systematic review application. Title and abstract screenings were performed independently by 2 reviewers (ICV and MP) to verify study eligibility and remove the remaining duplicates. Conflicting decision(s) regarding study eligibility were resolved by discussion with a content expert (DKW). A full-text review was performed prior to the data extraction whenever necessary.- 3. Data extraction and analysis
- 3. Data extraction and analysis
Study characteristics (year of publication, first author, country of origin, country income group, study design, setting, objective (s), inclusion and exclusion criteria, study period, number of subjects, age, and sex ratio) were extracted and tabulated. Continent and country were collated according to the study subjects’ country of origin. The country income group was defined according to World Bank classification [20]. Age categories (upper age limit for neonates, 1 month; infants, 2 years; children, 12 years; adolescents, 18 years) were defined based on the baseline characteristics and/or study eligibility criteria [21]. Data related to ferritin measurement (definition, threshold, diagnostic test indices, normal range, proportion, summary statistics) and predicted outcomes (diagnosis or condition, outcome, significance, statistical analysis) were iteratively tabulated to obtain comprehensive data.A symbol map was created using Tableau Public that can be accessed at https://public.tableau.com/views/FerritinMap/Dashboard1?:language=en-US&publish=yes&:display_count=n&:origin=viz_share_link . Data visualization using horizontal boxplots was performed in Python 3.9.7 using the matplotlib plotting library. The central tendencies were plotted as a vertical line between whiskers representing the upper and lower values of dispersion. Logarithmic transformation with a base of 10 was performed for scaling purposes while retaining the original absolute serum ferritin levels. Hence, negative values of the lower bound of the lower whisker in instances in which the standard deviation was greater than the mean were omitted considering that the actual value should always be positive.
This scoping review followed a previously established methodological framework [16,17] according to the Preferred Reporting Items for Systematic reviews and Meta-Analyses extension for Scoping Reviews checklist [18]. The a priori study protocol was followed closely to ensure a transparent and systematic approach [19].
- Results
- Results
- 1. Summary statistics
- 1. Summary statistics
Statistics for overall and condition-specific serum ferritin levels were reported by 30 (45.4%) and 47 (71.2%) of the studies, respectively (Table 2) [22-78]. Although most summary statistics were presented as median (interquartile range) (43.9%) or mean±standard deviation (43.9%), the remaining 12.1% were presented as median with or without range and mean with 95% confidence interval (CI). Baseline values (43.6%) were obtained more frequently than peak values (15.1%) and postintervention values (1.5%).The overall ferritin summary statistics are shown in Fig. 2. The ranges of overall serum ferritin levels in hospitalized patients, febrile seizures, heart failure (HF), idiopathic pulmonary hemosiderosis (IPH), and very low birth weight (VLBW) infants largely spanned tens and hundreds of nanograms per milliliter. While the ranges of overall serum ferritin in COVID-19, sepsis and septic shock vary widely from hundreds to thousands, their upper extremes coincide with those of MIS-C, scrub typhus, and pediatric multisystem inflammatory syndrome temporally associated with severe acute respiratory syndrome coronavirus-2 (SARS-CoV-2) mimicking Kawasaki disease (KD; KawaCOVID-19). The overall range for hemophagocytic lymphohistiocytosis (HLH) was gauged at thousands in contrast with the broadly extending ranges of macrophage activation syndrome (MAS) and overlap of secondary HLH/MAS, which extends from thousands to tens of thousands. Cytokine release syndrome had the highest range, encompassing tens of thousands to hundreds of thousands of serum ferritin (in ng/mL).Serum ferritin levels in the case and intervention groups are presented in Fig. 3. The ranges for complex febrile seizure and extremely low birth weight infants were within tens of nanograms per milliliters. The ranges gradually shifted to the right within hundreds in very preterm, very preterm, and VLBW infants, overarched by neonates requiring resuscitation. Respiratory conditions such as bronchiolitis, IPH, and Mycoplasma pneumoniae pneumonia (MPP) with hypoxia and sepsis spectrum were within hundreds of nanograms per milliliter, while adenoviral pneumonia along with fulminant Wilson disease and scrub typhus showed greater values at thousands of nanograms per milliliter. Data on dispersion were not available for MPP, and the central tendencies of refractory MPP and MPP with hypoxia were far apart. The figure for COVID-19 spread across hundreds to thousands, which overlapped MIS-C, with the exception of further extension to tens of thousands in the latter. Systemic juvenile idiopathic arthritis (sJIA) before and after MAS, MAS in KD, and Kawa-COVID-19, although inconsistently, spanned thousands of serum ferritin levels. Notably, primary and secondary HLH and dengue-associated HLH extended from thousands to hundreds of thousands.- 2. Normal ranges and thresholds
- 2. Normal ranges and thresholds
The normal range of serum ferritin level was defined in 16 studies (24.2%), while its threshold was predetermined in 32 studies (48.5%) and determined by analysis in 11 studies (16.7%). A value of less than 500 ng/mL was most often considered the upper limit of normal (5 of 16 [31.2%]) and threshold (11 of 43 [25.6%]). Only 2 studies specified demographic-specific normal range values. A wide variation in serum ferritin threshold was observed within and between different conditions or diseases of interest. The proportions of increased, normal, and decreased serum ferritin levels were reported in 25 (37.9%), 6 (9.1%), and 7 studies (10.6%), respectively. Iron status was more often defined as a decreased serum ferritin level (57.1%) than an increased serum ferritin level (4%). An increased serum ferritin was used to indicate hyperferritinemia (16%), and the diagnosis or disease activity (12%) was almost equal (Table 3) [75-87].- 3. Predictive ability
- 3. Predictive ability
Among the 55 studies (83.3%) analyzing serum ferritin predictive ability against outcomes, 45 (81.8%) yielded significant results. Thirty of 38 analyses (78.9%) treated serum ferritin as a numerical variable, while 11 of 13 analyses (84.6%) treated it as a categorical variable with a preset threshold. Serum ferritin levels in the case or intervention group were generally (80.8%) higher than those in the control or comparator group. A significant relationship was found with mortality (12 of 46 [26.1%]), morbidity (18 of 46 [39.1%]), and specific (16 of 46 [34.8%]) outcomes in ICU patients (3,000 ng/mL), VLBW infants (500 ng/mL), febrile seizure, HF secondary to dilated cardiomyopathy, IPH, adenoviral pneumonia, MPP (171.15 and 174.15 ng/mL), COVID-19 in pediatric oncology (1,000 ng/mL) and inborn errors of immunity, HLH (10,000 ng/mL), KD, KawaCOVID-19, MAS (50,000 ng/mL), sJIA, MIS-C (500 ng/mL and per 100-unit increase), sepsis (300 and 500 ng/mL), severe sepsis, dengue-associated HLH (500 ng/mL), and allogeneic hematopoetic cell transplantation (1,000 ng/mL). Diagnostic test indices reported as area under the curve (AUC; 95% CI), sensitivity, and specificity achieved significance in hospitalized patients (373 ng/mL; AUC, 0.88; 95% CI, 0.79–0.97), Wilson disease (93.5 ng/mL; 95% CI, 0.773–0.989; 92.9%, 66.2%), MPP with hypoxia (171.15 ng/mL; AUC, 0.806; 82.4%, 69.3%), and refractory (230 ng/mL; 67%, 67%), Kawa-COVID-19 (1,400 ng/mL; AUC, 0.957; 80%, 100%), sepsis (135 ng/mL; AUC, 0.785; 95% CI, 0.733–0.830; 96%, 49%; 500 ng/mL; AUC, 0.767; 95% CI, 0.633–0.900); 1,210 ng/mL; AUC, 0.89; 95% CI, 0.74–1.04; 88%, 85%), severe sepsis (500 ng/mL; 64%, 80%; 1,980 ng/mL; AUC, 0.878; 95% CI, 0.751–1.000; 75%, 92%), and HLH (10,000 ng/mL; AUC, 0.92; 95% CI, 0.88–0.95; 90%, 96%) (Supplementary Material 2).
A total of 66 of the 1,580 identified citations were included in the current scoping review (Fig. 1). Complete information about the included studies can be found in Supplementary Material 2. The exponential growth in the number of studies on ferritin has been evident in the last 2 decades. Up to 36.4% of the included studies were published in 2021. Observational (56.1%) and cohort (22.7%) study designs were predominant, while the minority of others (3.0%) consisted of survey and surveillance studies. The number of retrospective studies (50.0%) slightly exceeded that of their prospective counterparts (37.9%). The study settings were as follows: hospital (36.3%), intensive care unit (ICU) (27.3%), multicenter (24.2%) to university facilities (6.1%), and clinics (3.0%). Of the 34 representative countries, most studies were performed in Asia (45.6%), and underrepresentation of Africa (1.5%) was noteworthy. Lower- to middleincome countries were represented by only 14 studies (21.2%) (Table 1).Authors from the United States (16 [24.2%]), India (9 [13.6 %]), and Turkey (7 [10.6%]) generated nearly half of the studies, whereas most subjects originated from the United States (2,837 [32.4%]), Pakistan (971 [11.1%]), and India (925 [10.6%]) (Supplementary Material 3). More than half (63.6%) of the studies involved fewer than 100 subjects per study. Nonetheless, one multicenter surveillance study involved 1,080 subjects across the United States. Children constituted the largest proportion (77.2%) of the study subjects, even more than the mix of more than 2 pediatric age categories (43.9%). A male preponderance was observed in 48 of 63 studies (76.2%) (Table 1).
- Discussion
- Discussion
- 1. Outcome predicting thresholds
- 1. Outcome predicting thresholds
Considering the statistical significance and baseline serum ferritin measurements, thresholds for predicting hypoxia in MPP (174.15 ng/mL) [31], severe acute kidney injury (AKI) in MIS-C (per 100-unit increase) [62], fewer ventilation-free hours and higher maximum inotropic score (300 ng/mL) [67] and HLH (500 ng/mL) [65] in sepsis, mortality in severe sepsis (1,980 ng/mL) [85], and stage-3 AKI in allogeneic hematopoietic cell transplantation (1,000 ng/mL) [86] are outcome-defining thresholds for predictions upon admission. Peak serum ferritin thresholds for surgical ligation of patent ductus arteriosus, sepsis, and moderate or severe states of bronchopulmonary dysplasia in VLBW infants (500 ng/mL) [80], DNA viremia in sepsis (1,210 ng/mL) [64], and mortality in hospitalized patients (373 ng/mL) [22], ICU admitted patients (3,000 ng/mL) [87], and HLH (10,000 ng/mL) [41] are more suitable for monitoring purposes.- 2. Study characteristic representation
- 2. Study characteristic representation
Descriptive analysis revealed the underrepresentation of several key demographic features. Age and sex cause significant differences in the serum ferritin reference range, even within an apparently healthy pediatric population [93,94]. Most reviewed studies included mixed age categories and predominantly included men. Appreciation of these features was considered in only 2 studies that provided age- and sex-specific normal ranges. Meanwhile, no such specification has been reported for serum ferritin thresholds. The disparity in studies’ countries of origin has 2 indirect implications, namely, ethnicity and country income. While ethnicity-related genetic variants are reportedly associated with serum ferritin differences [95], the paucity of data from lowerto middle-income countries can lead to disease burden underestimation, thus impeding diagnostic and therapeutic improvement in resource-limited settings where it is needed the most. These issues can be addressed by adjusting the key demographic features according to study design or analysis and specific reporting of serum ferritin levels.- 3. Methods and translation into clinical praxis
- 3. Methods and translation into clinical praxis
The first concern was the wide range of serum ferritin levels that were evident between conditions as well as studies on the same condition. Nevertheless, this phenomenon is readily observed in normally distributed data that reported a standard deviation greater than the mean. The skewed normal distribution is a plausible explanation for this finding, which was at least partially due to the small sample size. This was confirmed in the current review, in which 63.6% of studies included fewer than 100 subjects. Skewness rendered the mean and standard deviation unrepresentative of the actual central tendency and dispersion [96]. Hence, improved precision by increasing of the sample size in future studies is imperative to generate the true serum ferritin threshold.Unjustified thresholds (74.4%) were 3 times more common than justified thresholds (25.6%). Even among those justified by the analysis, methods to determine optimal threshold values were mentioned in only 3 of 11 studies (27.3%). All 3 studies used the Youden index derived from the sum of the sensitivity and specificity subtracted by 100%. The importance of justifying the methods cannot be overstated, especially in critical conditions when decision-making strategies become the cornerstone of the outcome. Various approaches are available to determine the optimal threshold from the receiver operating characteristic analysis [97]. Furthermore, the integration of outcome probability, cost of misprediction, and patients’ values and preferences in advanced modeling can accelerate the substantiation of evidence-based clinical practice.- 4. Mechanistic underpinnings
- 4. Mechanistic underpinnings
Finally, the vast growth of serum ferritin diagnostic studies requires balancing with a sound understanding of the underlying clinicopathological mechanisms. This is particularly crucial because serum ferritin level is highly dynamic and not exclusively unidirectional (i.e., decrease or increase); rather, it can change in both directions simultaneously, although it usually increases to a greater extent in the context of critical illness. Anemia of inflammation in critical illness is an excellent example of how such concurrent changes can lead to underperformance of positive acute-phase reactants such as serum ferritin level [98]. The net change in serum ferritin level is an outward manifestation stemming from normal and pathological pathways that warrants further study [99]. Understanding the mechanistic underpinnings allows for better clinical conditions and/or outcome targets.- 5. Strengths and limitations
- 5. Strengths and limitations
To the best of our knowledge, this is the first systematic scoping review of ferritin levels in critical pediatric illness. We used an a priori defined protocol with specific eligibility criteria sufficient to limit unnecessary information but still allowed comprehensive thematic scoping. However, our study was limited by its lack of access to subscription-based databases, which may have narrowed our search results and unintentionally excluded relevant studies.In conclusion, despite rapidly growing evidence about the predictive ability of ferritin level, its translation to clinical practice is still hampered by underrepresentation of demographic features, inadequate sample size, and lack of methodological rigor and understanding of underlying pathways. Future directions include increased precision in serum ferritin measurements followed by close attention to the threshold modeling strategy and reporting.
The increasing burden of pediatric critical illness necessitates advancements in mortality and morbidity outcome predictions [88-91]. A growing body of evidence supports the potential use of ferritin as a biomarker in multiple clinical contexts. However, without careful interpretation, its equivocal nature can inevitably confound its predictive ability [92]. Therefore, this study aimed to identify the prospects and gaps in interpreting serum ferritin levels in different clinical conditions. A total of 22 predictive thresholds for 15 unique conditions were identified.
Supplementary material
Supplementary material
Supplementary materials: Supplementary materials 1-3 can be found via https://doi.org/10.3345/cep.2022.00654.Supplementary Material. 3.
cep-2022-00654-Supplementary-Material-3.pdfSymbol map showing country of origin, number of studies (color scale), and number of study subjects (circular area and label).
- Footnotes
-
Conflict of interest No potential conflict of interest relevant to this article was reported.
Funding This study received no specific grant from any funding agency in the public, commercial, or not-for-profit sectors.
-
Fig. 2.
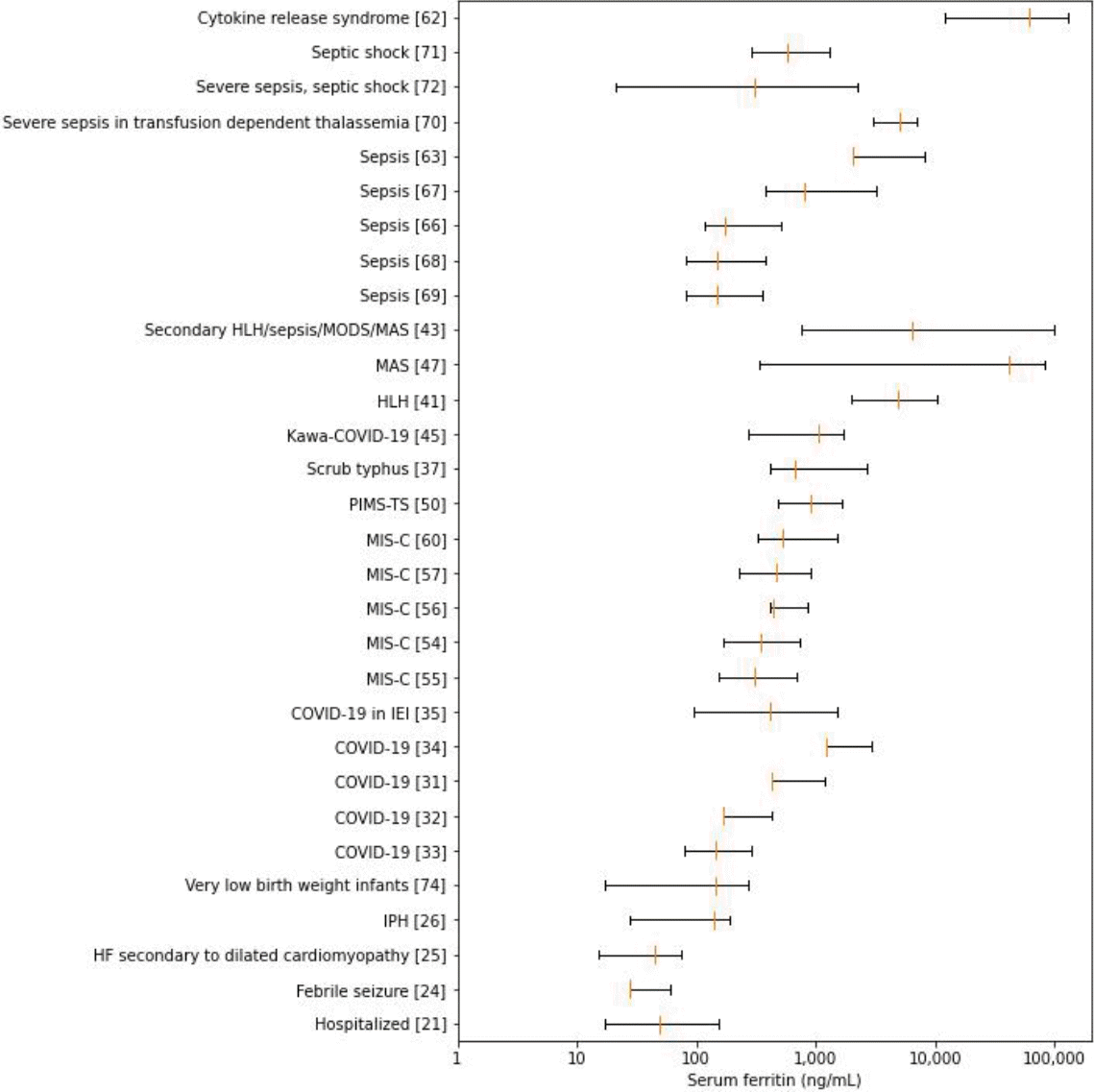
Fig. 3.
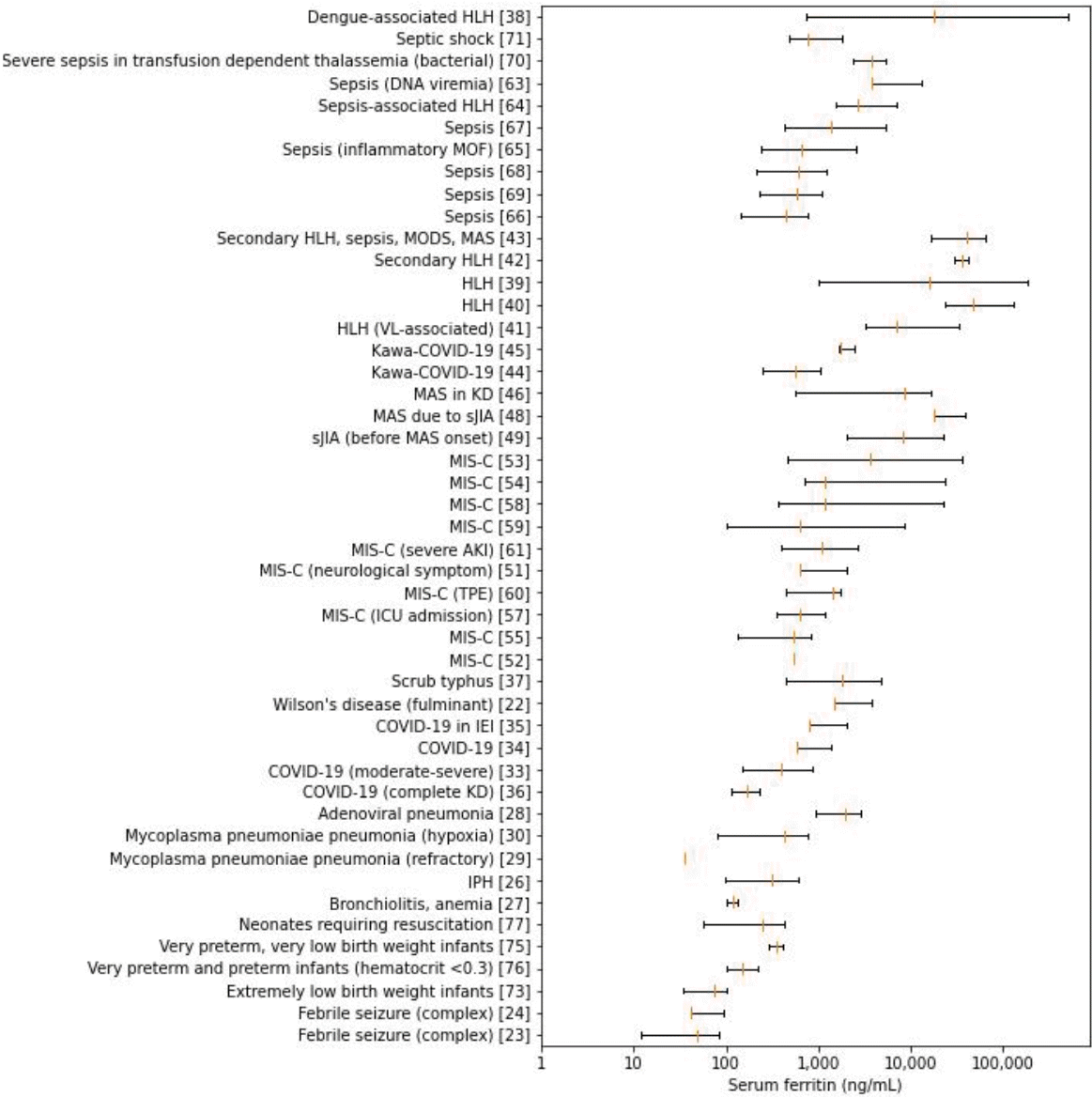
Table 1.
Characteristic | Number (%) |
---|---|
Year of publication | |
2018–2021 | 45 (68.2) |
2013–2017 | 12 (18.2) |
2008–2012 | 5 (7.6) |
2003–2007 | 4 (6.1) |
Continenta) | |
Asia | 31 (45.6) |
America | 23 (33.8) |
Europe | 13 (19.1) |
Africa | 1 (1.5) |
Country income groupa) | |
High | 40 (58.8) |
Upper middle | 14 (20.6) |
Lower middle | 14 (20.6) |
Design | |
Observational | 37 (56.1) |
Cohort | 15 (22.7) |
Case control | 6 (9.1) |
Clinical trial | 6 (9.1) |
Others | 2 (3.0) |
Type | |
Retrospective | 33 (50.0) |
Prospective | 25 (37.9) |
Combined | 3 (4.5) |
Cross-sectional | 2 (3.0) |
Not given | 3 (4.5) |
Setting | |
Hospital | 24 (36.3) |
Intensive care unit | 18 (27.3) |
Multicenter | 16 (24.2) |
Others | 4 (6.1) |
Clinic | 2 (3.0) |
Not given | 2 (3.0) |
No. of subjects | |
<100 | 42 (63.6) |
100–1,000 | 23 (34.8) |
>1,000 | 1 (1.5) |
Age category | |
Mixed | 29 (43.9) |
Infants and children | 12 (18.2) |
Children | 10 (15.1) |
Adolescents | 8 (12.1) |
Neonates | 7 (10.6) |
Table 2.
Study | Overall | Case/intervention | Control/comparator | Condition |
---|---|---|---|---|
Horvat [22] | 49 (17.2–153.3)p) | - | - | Hospitalized |
Güngör [23] | - | 1,503.50±2,280.44 | 57.43±35.96 | Wilson disease |
Papageorgiou [24]* | - | 48.61±36.63 | 74.03±38.04 | Febrile seizure |
Turay [25]* | 28±32 | 41±54 | 22±16 | Febrile seizure |
Higgins [26]* | 45±30 | - | - | HF secondary to dilated cardiomyopathy |
Yang [27]* | 141.3(27.9–188.0) | 320 (98.8–619) | 38.7 (12.7–60.2) | IPH |
Jacobs [28] | - | 117.6±16.2 | 134.4±27.6 | Bronchiolitis, anemia |
Xu [29]* | - | 1,945±1,001 | 465±195 | Adenoviral pneumonia |
Choi [30]* | - | 35.95a) | - | Mycoplasma pneumoniae pneumonia |
Ling [31] | - | 421.61±341.06 | 150.85±167.70 | Mycoplasma pneumoniae pneumonia |
Alshengeti [32] | 424.35±763.85 | - | - | COVID-19 |
Astagimath [33] | 167.2±256.6 | - | - | COVID-19 |
Jat [34] | 144 (80.1–290) | 397.9 (148–879.2) | 132 (80.1–196) | COVID-19 |
Alfraij [35] | 1,229±1,689 | 587±796 | 1,358±1,807 | COVID-19 |
Karimi [36]* | 413 (95–1,500) | 800 (800–2,037) | 125 (78–413) | COVID-19 in IEI |
Clark [37]* | - | 171±57 | 678±107 | COVID-19 |
Williams [38] | 668 (420–2,714) | 1,806 (443–4,727) | 666 (382–2,653) | Scrub typhus |
Ellis [39] | - | 17,794 (754–522,000)a) | 4,139 (42–30,346) | Dengue |
Allen [40] | - | 15,830 (994–189,721)a,c) | - | HLH |
Goldman [41] | - | 47,500 (24,016–129,604)c) | 11,884 (3,130–36,602)c) | HLH |
Mottaghipisheh [42]* | 4,825 (2,000.0–10,324.5) | 7,122 (3,226.2–33,112.2)a) | 3,618 (1,990.2–9,479.2) | HLH |
Cui [43] | - | 35,803±5,826 | 36,290±6,205 | Secondary HLH |
Demirkol [44] | 6,341 (765–100,000) | 40,802 (16,348–65,256)a) | 5,054 (765–100,000) | Secondary HLH, sepsis, MODS, MAS |
Cattalini [45]* | - | 563.8 (250–1,068) | 227 (147–449) | KD |
Pouletty [46]* | 1,067 (272–1,709) | 1,760 (1,693–2,500) | 295 (165–536) | Kawa-COVID-19 |
Jinkawa [47] | - | 8,741±8,181.5 | - | MAS in KD |
Pal [48] | 41,785±41,448 | - | - | MAS |
Aydin [49]* | - | 17,863±21,846 | 753±586 | MAS due to sJIA, MIS-C |
Minoia [50]* | - | 8,235 (2,048–22,977) | 900 (171–2,968) | sJIA |
Penner [51] | 915 (475–1,650) | - | - | PIMS-TS |
Sa [52] | - | 640 (1,400)c) | 549 (871)c) | MIS-C |
Lee [53]* | - | 537a) | 4,594 | MIS-C |
Pereira [54]* | - | 3,660 (469–35,976)a) | 3,295 (2,567–8,000) | MIS-C |
Tiwari [55]* | 350 (170–733)c) | 1,178.0 (717.0–23,840.0)c) | 266.0 (153.0–555.0)c) | MIS-C |
Torres [56] | 309 (156–696) | 542 (135–835) | 230 (156–298) | MIS-C |
Valverde [57]* | 438 (420–846) | - | - | MIS-C |
Abrams [58]* | 463 (225–911)c) | 626 (350–1,171)c) | 289 (156–529)c) | MIS-C |
Atay [59]* | - | 1,200 (367–22,627) | 332.5 (16–3,274) | MIS-C |
Klymet [60]* | - | 625 (103–8,433) | 310 (48.5–5,546) | MIS-C |
Emeksiz [61]* | 535 (326.8–1,533.1) | 1,435 (445.5–1,744) | 438.5 (226.3–671.5) | MIS-C |
Deep [62]* | - | 1,107 (393–2,668) | 426 (209–958) | MIS-C |
Fitzgerald [63] | 60,214 (12,000–130,000)c) | - | - | Cytokine release syndrome |
Simon [64]* | 2,025±6,233c) | 3,752±9,245c) | 874±2,369c) | Sepsis |
Cui [65]* | - | 2,689 (1,554–7,142) | 1,025.2 (682.2–1,560) | Sepsis |
Carcillo [66]* | - | 670 (240–2,610), 885 (285–3,205), 1,100 (390–4,770)c) | 200 (120–420), 185 (100–360)c) | Sepsis |
Tonial [67]* | 172 (118.3–514) | 454.4±309.7 | 91.9±6 | Sepsis |
Williams [68] | 798 (378–3,205) | 1,381 (433–5,410) | 707 (365–2,937) | Sepsis |
Tonial [69]* | 149.5 (81.7–377.2)c) | 607 (217–1,251)c) | 138 (78–296)c) | Sepsis |
Tonial [70]* | 150.5 (82.25–362) | 586 (226–1,093) | 138 (80.5–287) | Sepsis |
Rasheed [71]* | 4,989.3±1,978.3 | 3,849.5±1,513.5 | 6,413.5±2,103.9 | Severe sepsis in transfusion dependent thalassemia |
Ghosh [72] | 581 (287–1,283) | 763 (480–1,820) | 415 (262–852) | Septic shock |
Garcia [73]* | 303 (21–2,210) | - | - | Severe sepsis, septic shock |
Haiden [74] | - | 73.6 (34.3–99.9) | 97.5 (60.8–126.0) | Extremely low birth weight infants |
Al-Ghananim [75] | 143±126 | - | - | Very low birth weight infants |
Widness [76] | - | 351±55 | 310±32 | Very preterm, very low birth weight infants |
Mäkelä [77] | - | 148.2 (100.7–218.2)b) | 239.5 (135.2–424.3) | Very preterm and preterm infants |
Ram Mohan [78] | - | 244.853±187.334d) | 148.542±161.961d) | Neonates requiring resuscitation |
HF, heart failure; IPH, idiopathic pulmonary hemosiderosis; COVID-19, coronavirus disease 2019, IEI, inborn errors of immunity; HLH, hemophagocytic lymphohistiocytosis; MODS, multiple organ dysfunction syndrome; AKI, acute kidney injury; MAS, macrophage activation syndrome; KD, Kawasaki disease; sJIA, systemic juvenile idiopathic arthritis; MIS-C, multisystem inflammatory syndrome in children; PIMS-TS, pediatric inflammatory multisystem syndrome temporally associated with severe acute respiratory syndrome coronavirus-2.
Table 3.
Study | Threshold (ng/mL) (normal range) |
Proportion |
Condition | ||
---|---|---|---|---|---|
Increased (%) | Normal (%) | Decreased (%) | |||
Al-Ghananim [75] | 15 | - | 100 | - | Very low birth weight infants |
Widness [76] | 15 | - | 38.9 | Very preterm, very low birth weight infants | |
Amin [79] | 76, 400 (76–400) | 18.6 | 53.6 | 21.4 | Very preterm infants |
Ochiai [80]* | 500 | 13.6 | - | - | Very low birth weight infants |
Papageorgiou [24] | 30 | - | - | 14 | Febrile seizure |
Güngör [23] | 93.5a) (31–99) | - | - | - | Wilson’s disease |
Higgins [26] | 100 | - | - | 92.9 | HF secondary to dilated cardiomyopathy |
Ling [31]* | 171.15 (ROC), 174.15 (logistic regression)a) | - | - | - | Mycoplasma pneumoniae pneumonia |
Choi [30] | 230a) | - | - | - | Mycoplasma pneumoniae pneumonia |
Horvat [22] | 373a) | - | - | - | Hospitalized |
Astagimath [33] | (male, 24–336; female, 11–307) | - | - | - | COVID-19 |
Jat [34] | 60 (≤9 years), 300 (10–12 years) | 58.9 | - | - | COVID-19 |
Alshengeti [32] | 500 (<500) | - | 85.7 | 14.3 | COVID-19 |
Fernandes [81] | 500 | 51.2 | - | - | COVID-19, MIS-C |
Hammad [82]* | 1,000 | 67.6 | - | - | COVID-19 in pediatric oncology |
Pereira [54] | (36–91) | - | - | - | MIS-C |
Emeksiz [61] | 140 (7–140) | - | - | - | MIS-C |
Lee [53] | 200 | 86 | - | - | MIS-C |
Valverde [57] | 200 (50–200) | 79 | - | - | MIS-C |
Penner [51] | 300 (15.1–70.0) | - | - | - | PIMS-TS |
Tiwari [55] | 300 | 54 | - | - | MIS-C |
Balagurunathan [83]* | 500 | 42.9 | - | - | MIS-C |
Acevedo [84]* | 500 | 50 | - | - | MIS-C |
Deep [62]* | Per 100–unit increasea) | - | - | - | MIS-C |
Ellis [39]* | 500 | 62.7 | - | - | Dengue |
Mottaghipisheh [42] | 500 | 100 | - | - | HLH |
Demirkol [44] | 500 | 100 | - | - | Secondary HLH/sepsis/MODS/MAS |
Goldman [41]* | 10,000 | - | - | - | HLH |
Allen [40] | 10,000 | - | - | - | HLH |
Tonial [69] | 135a) | 40 | - | - | Sepsis |
Tonial [67]* | 300 | - | - | - | Sepsis |
Cui [65]* | 500 | - | - | - | Sepsis |
Williams [68] | 500 (≤500) | 67.8 | 32.2 | - | Sepsis |
Simon [64] | 1,210a) (≤1,000) | 38.9 | - | - | Sepsis |
Carcillo [85] | 1,980a) | 13 | - | - | Severe sepsis |
Garcia [73] | 500 (200–500) | 33.3 | 30.6 | 36.1 | Severe sepsis, septic shock |
Ghosh [72] | 200 (50–300) | 63.9 | - | 36.1 | Septic shock |
Minoia [50] | (18–300) | - | - | - | sJIA |
Aydin [49] | 684 | - | - | - | MAS due to sJIA, MIS-C |
Jinkawa [47] | 684 | 100 | - | - | MAS in KD |
Pal [48]* | 50,000 (10–118) | - | - | - | MAS |
Kurokawa [86]* | 1,000 | 37.7 | - | - | Allogeneic HCT |
Pouletty [46] | 1,400a) | 85.7 | - | - | Kawa-COVID-19 |
Williams [38] | 2,000a) (<500) | 72.6 | 27.4 | - | Scrub typhus |
Bennett [87]* | 3,000 | 39.8 | - | - | ICU |
Fitzgerald [63] | 4,984.4a) (≤500) | 46 | - | - | Cytokine release syndrome |
HF, heart failure; COVID-19, coronavirus disease 2019; MIS-C, multisystem inflammatory syndrome in children; PIMS-TS, pediatric inflammatory multisystem syndrome temporally associated with severe acute respiratory syndrome coronavirus-2; HLH, hemophagocytic lymphohistiocytosis; MODS, multiple organ dysfunction syndrome; MAS, macrophage activation syndrome; sJIA, systemic juvenile idiopathic arthritis; KD, Kawasaki disease; HCT, hematopoietic cell transplantation; ICU, intensive care unit.
- References
- 1. Crichton R. Biomineralization. Crichton R, editor. Biological inorganic chemistry. 3rd ed. London: Elsevier, 2019;:517–544.2. Wang W, Knovich MA, Coffman LG, Torti FM, Torti SV. Serum ferritin: past, present and future. Biochim Biophys Acta 2010;1800:760–9.
[Article] [PubMed] [PMC]3. Mahroum N, Alghory A, Kiyak Z, Alwani A, Seida R, Alrais M, et al. Ferritin - from iron, through inflammation and autoimmunity, to COVID-19. J Autoimmun 2022;126:102778.
[Article] [PubMed] [PMC]4. GBD 2017 Child and Adolescent Health Collaborators; Reiner RC Jr, Olsen HE, Ikeda CT, Echko MM, Ballestreros KE, et al. Diseases, injuries, and risk factors in child and adolescent health, 1990 to 2017: findings from the global burden of diseases, injuries, and risk factors 2017 study. JAMA Pediatr 2019;173:e190337.
[PubMed] [PMC]5. Zee-Cheng JE, McCluskey CK, Klein MJ, Scanlon MC, Rotta AT, Shein SL, et al. Changes in pediatric ICU utilization and clinical trends during the coronavirus pandemic. Chest 2021;160:529–37.
[Article] [PubMed] [PMC]6. Paediatric Intensive Care Audit Network. Annual report 2021. PICANet 2021 Report: data collection period January 2018–December 2020 [Internet]. Leeds (UK), Paediatric Intensive Care Audit Network. c2022;[cited 2022 Apr 1]. Available from: https://www.picanet.org.uk/wpcontent/uploads/sites/25/2022/01/PICANet-2021-Annual-Report_v1.0-13Jan2022-2.pdf.7. Murphy Salem S, Graham RJ. Chronic illness in pediatric critical care. Front Pediatr 2021;9:686206.
[Article] [PubMed] [PMC]8. Procter C, Morrow B, Pienaar G, Shelton M, Argent A. Outcomes following admission to paediatric intensive care: A systematic review. J Paediatr Child Health 2021;57:328–58.
[Article] [PubMed]9. Zimmerman JJ, Anand KJ, Meert KL, Willson DF, Newth CJ, Harrison R, et al. Research as a standard of care in the PICU. Pediatr Crit Care Med 2016;17:e13–21.
[Article] [PubMed] [PMC]10. Garcia-Casal MN, Pasricha SR, Martinez RX, Lopez-Perez L, Peña-Rosas JP. Serum or plasma ferritin concentration as an index of iron deficiency and overload. Cochrane Database Syst Rev 2021;5:CD011817.
[Article] [PubMed] [PMC]11. Ramírez-Carmona W, Díaz-Fabregat B, Yuri Yoshigae A, Musa de Aquino A, Scarano WR, de Souza Castilho AC, et al. Are serum ferritin levels a reliable cancer biomarker? A systematic review and meta-analysis. Nutr Cancer 2022;74:1917–26.
[Article] [PubMed]12. Melo AKG, Milby KM, Caparroz ALMA, Pinto ACPN, Santos RRP, Rocha AP, e al. Biomarkers of cytokine storm as red flags for severe and fatal COVID-19 cases: a living systematic review and meta-analysis. PLoS One 2021;16:e0253894.
[Article] [PubMed] [PMC]13. Zhang M, Li W, Wang T, Zhang Q. Association between baseline serum ferritin and short-term outcome of intracerebral hemorrhage: a metaanalysis. J Stroke Cerebrovasc Dis 2019;28:1799–805.
[Article] [PubMed]14. Centers for Disease Control and Prevention. Multisystem inflammatory syndrome in children (MIS-C) associated with coronavirus disease 2019 (COVID-19) [Internet]. Atlanta (GA), Centers for Disease Control and Prevention. c2020;[cited 2022 Apr 30]. Available from: https://emergency.cdc.gov/han/2020/han00432.asp.15. Royal College of Paediatrics and Child Health. Guidance: paediatric multisystem inflammatory syndrome temporally associated with COVID-19 [Internet]. London, The Royal College of Paediatrics and Child Health. c2020;[cited 2022 Apr 30]. Available from: https://www.rcpch.ac.uk/sites/default/files/2020-05/COVID-19-Paediatric-multisystem-%20inflammatory%20syndrome-20200501.pdf.16. Arksey H, O’Malley L. Scoping studies: towards a methodological framework. Intl J Social Res Method 2007;8:19–32.
[Article]17. Levac D, Colquhoun H, O'Brien KK. Scoping studies: advancing the methodology. Implement Sci 2010;5:69.
[Article] [PubMed] [PMC]18. Tricco AC, Lillie E, Zarin W, O'Brien KK, Colquhoun H, Levac D, et al. PRISMA Extension for Scoping Reviews (PRISMA-ScR): checklist and explanation. Ann Intern Med 2018;169:467–73.
[Article] [PubMed]19. Valerie IC, Prabandari M, Wati DK. Ferritin in pediatric critical illness: a scoping review protocol [Internet]. Vancouver, Open Science Framework. 2022;[cited 2022 Apr 30]. Available from: https://osf.io/gzdrx.20. The World Bank. The world by income and region [Internet]. Washington, DC, The World Bank. c2021;[cited 2022 May 5]. Available from: https://datatopics.worldbank.org/world-development-indicators/the-world-by-income-and-region.htm.21. Williams K, Thomson D, Seto I, Contopoulos-Ioannidis DG, Ioannidis JP, Curtis S, et al. Standard 6: age groups for pediatric trials. Pediatrics 2012;129 Suppl 3:S153–60.
[Article] [PubMed]22. Horvat CM, Bell J, Kantawala S, Au AK, Clark RSB, Carcillo JA. C-reactive protein and ferritin are associated with organ dysfunction and mortality in hospitalized children. Clin Pediatr (Phila) 2019;58:752–60.
[Article] [PubMed] [PMC]23. Güngör Ş, Selimoğlu MA, Varol Fİ, Güngör S, Üremiş MM. The effects of iron and zinc status on prognosis in pediatric Wilson's disease. J Trace Elem Med Biol 2019;55:33–8.
[Article] [PubMed]24. Papageorgiou V, Vargiami E, Kontopoulos E, Kardaras P, Economou M, Athanassiou-Mataxa M, et al. Association between iron deficiency and febrile seizures. Eur J Paediatr Neurol 2015;19:591–6.
[Article] [PubMed]25. Turay S, Hanci F, Ozde S. An overview of vitamin B12 and iron deficiencies as a risk factors in children with febrile seizure etiology. Exp Biomed Res 2021;4:154–63.
[Article]26. Higgins D, Otero J, Jefferis Kirk C, Pak J, Jorgensen N, Kemna M, et al. Iron laboratory studies in pediatric patients with heart failure from dilated cardiomyopathy. Am J Cardiol 2017;120:2049–55.
[Article] [PubMed]27. Yang CT, Chiang BL, Wang LC. Aggressive corticosteroid treatment in childhood idiopathic pulmonary hemosiderosis with better outcome. J Formos Med Assoc 2021;120:838–46.
[Article] [PubMed]28. Jacobs BR, Lyons K, Brilli RJ. Erythropoietin therapy in children with bronchiolitis and anemia. Pediatr Crit Care Med 2003;4:44–8.
[Article] [PubMed]29. Xu N, Chen P, Wang Y. Evaluation of risk factors for exacerbations in children with adenoviral pneumonia. Biomed Res Int 2020;2020:4878635.
[Article] [PubMed] [PMC]30. Choi YJ, Jeon JH, Oh JW. Critical combination of initial markers for predicting refractory Mycoplasma pneumoniae pneumonia in children: a case control study. Respir Res 2019;20:193.
[Article] [PubMed] [PMC]31. Ling Y, Zhang T, Guo W, Zhu Z, Tian J, Cai C, et al. Identify clinical factors related to Mycoplasma pneumoniae pneumonia with hypoxia in children. BMC Infect Dis 2020;20:534.
[Article] [PubMed] [PMC]32. Alshengeti A, Alahmadi H, Barnawi A, Alfuraydi N, Alawfi A, Al-Ahmadi A, et al. Epidemiology, clinical features, and outcomes of coronavirus disease among children in Al-Madinah, Saudi Arabia: a retrospective study. Int J Pediatr Adolesc Med 2022;9:136–42.
[Article] [PubMed] [PMC]33. Astagimath MN, Raviraja A, Doddamani S. Biochemical and inflammatory markers in COVID-19 patients of a tertiary care hospital at North Karnataka – a descriptive study. J Stress Physiol Biochem 2021;17:105–12.34. Jat KR, Sankar J, Das RR, Ratageri VH, Choudhary B, Bhat JI, et al. Clinical profile and risk factors for severe disease in 402 children hospitalized with SARS-CoV-2 from India: Collaborative Indian Pediatric COVID Study Group. J Trop Pediatr 2021;67:fmab048.
[Article] [PubMed] [PMC]35. Alfraij A, Bin Alamir AA, Al-Otaibi AM, Alsharrah D, Aldaithan A, Kamel AM, et al. Characteristics and outcomes of coronavirus disease 2019 (COVID-19) in critically ill pediatric patients admitted to the intensive care unit: a multicenter retrospective cohort study. J Infect Public Health 2021;14:193–200.
[Article] [PubMed] [PMC]36. Karimi A, Shokri Y, Jamee M, Heidari A, Nazarpack F, Fallahi M, et al. Clinical course and characteristics of COVID-19 in patients with inborn errors of immunity: a retrospective multicenter experience from Iran. Res Sq [Preprint]. Res Sq [Preprint]. 2021 [cited 2022 May 5]. Available from: https://www.researchsquare.com/article/rs-957348/v1.37. Clark BC, Sanchez-de-Toledo J, Bautista-Rodriguez C, Choueiter N, Lara D, Kang H, et al. Cardiac abnormalities seen in pediatric patients during the SARS-CoV2 pandemic: an international experience. J Am Heart Assoc 2020;9:e018007.
[PubMed] [PMC]38. Williams V, Menon N, Bhatia P, Biswal M, Sreedharanunni S, Jayashree M, et al. Hyperferritinemia in children hospitalized with scrub typhus. Trop Med Health 2021;49:15.
[Article] [PubMed] [PMC]39. Ellis EM, Sharp TM, Pérez-Padilla J, González L, Poole-Smith BK, Lebo E, et al. Incidence and risk factors for developing dengue-associated hemophagocytic lymphohistiocytosis in Puerto Rico, 2008-2013. PLoS Negl Trop Dis 2016;10:e0004939.
[Article] [PubMed] [PMC]40. Allen CE, Yu X, Kozinetz CA, McClain KL. Highly elevated ferritin levels and the diagnosis of hemophagocytic lymphohistiocytosis. Pediatr Blood Cancer 2008;50:1227–35.
[Article] [PubMed]41. Goldman J, Desai MS, McClain KL, Tcharmtchi MH, Kennedy CE, Thompson K, et al. Hepatobiliary dysfunction and disseminated intravascular coagulation increase risk of mortality in pediatric hemophagocytic lymphohistiocytosis. Pediatr Crit Care Med 2018;19:e522–30.
[Article] [PubMed] [PMC]42. Mottaghipisheh H, Kalantar K, Amanati A, Shokripour M, Shahriari M, Zekavat OR, et al. Comparison of the clinical features and outcome of children with hemophagocytic lymphohistiocytosis (HLH) secondary to visceral leishmaniasis and primary HLH: a single-center study. BMC Infect Dis 2021;21:732.
[Article] [PubMed] [PMC]43. Cui Y, Zhang YC, Kang YL, Ren YQ, Miao HJ, Wang F. High-volume hemofiltration in critically ill patients with secondary hemophagocytic lymphohistiocytosis/macrophage activation syndrome: a prospective study in the PICU. Pediatr Crit Care Med 2016;17:e437–43.
[Article] [PubMed]44. Demirkol D, Yildizdas D, Bayrakci B, Karapinar B, Kendirli T, Koroglu TF, et al. Hyperferritinemia in the critically ill child with secondary hemophagocytic lymphohistiocytosis/sepsis/multiple organ dysfunction syndrome/macrophage activation syndrome: what is the treatment? Crit Care 2012;16:R52.
[Article] [PubMed] [PMC]45. Cattalini M, Della Paolera S, Zunica F, Bracaglia C, Giangreco M, Verdoni L, et al. Defining Kawasaki disease and pediatric inflammatory multisystem syndrome-temporally associated to SARS-CoV-2 infection during SARS-CoV-2 epidemic in Italy: results from a national, multicenter survey. Pediatr Rheumatol Online J 2021;19:29.
[Article] [PubMed] [PMC]46. Pouletty M, Borocco C, Ouldali N, Caseris M, Basmaci R, Lachaume N, et al. Paediatric multisystem inflammatory syndrome temporally associated with SARS-CoV-2 mimicking Kawasaki disease (Kawa-COVID-19): a multicentre cohort. Ann Rheum Dis 2020;79:999–1006.
[Article] [PubMed] [PMC]47. Jinkawa A, Shimizu M, Nishida K, Kaneko S, Usami M, Sakumura N, et al. Cytokine profile of macrophage activation syndrome associated with Kawasaki disease. Cytokine 2019;119:52–6.
[Article] [PubMed]48. Pal P, Bathia J, Giri PP, Roy M, Nandi A. Macrophage activation syndrome in pediatrics: 10 years data from an Indian center. Int J Rheum Dis 2020;23:1412–6.
[Article] [PubMed]49. Aydın F, Çelikel E, Ekici Tekin Z, Coşkun S, Sezer M, Karagöl C, et al. Comparison of baseline laboratory findings of macrophage activation syndrome complicating systemic juvenile idiopathic arthritis and multisystem inflammatory syndrome in children. Int J Rheum Dis 2021;24:542–7.
[Article] [PubMed]50. Minoia F, Davì S, Horne A, Demirkaya E, Bovis F, Li C, et al. Clinical features, treatment, and outcome of macrophage activation syndrome complicating systemic juvenile idiopathic arthritis: a multinational, multicenter study of 362 patients. Arthritis Rheumatol 2014;66:3160–9.
[Article] [PubMed]51. Penner J, Abdel-Mannan O, Grant K, Maillard S, Kucera F, Hassell J, et al. 6-month multidisciplinary follow-up and outcomes of patients with paediatric inflammatory multisystem syndrome (PIMS-TS) at a UK tertiary paediatric hospital: a retrospective cohort study. Lancet Child Adolesc Health 2021;5:473–82.
[Article] [PubMed]52. Sa M, Mirza L, Carter M, Carlton Jones L, Gowda V, Handforth J, et al. Systemic inflammation is associated with neurologic involvement in pediatric inflammatory multisystem syndrome associated with SARS-CoV-2. Neurol Neuroimmunol Neuroinflamm 2021;8:e999.
[Article] [PubMed] [PMC]53. Lee PY, Day-Lewis M, Henderson LA, Friedman KG, Lo J, Roberts JE, et al. Distinct clinical and immunological features of SARS-CoV-2-induced multisystem inflammatory syndrome in children. J Clin Invest 2020;130:5942–50.
[Article] [PubMed] [PMC]54. Pereira MFB, Litvinov N, Farhat SCL, Eisencraft AP, Gibelli MABC, Carvalho WB, et al. Severe clinical spectrum with high mortality in pediatric patients with COVID-19 and multisystem inflammatory syndrome. Clinics (Sao Paulo) 2020;75:e2209.
[Article] [PubMed] [PMC]55. Tiwari A, Balan S, Rauf A, Kappanayil M, Kesavan S, Raj M, et al. COVID-19 related multisystem inflammatory syndrome in children (MIS-C): a hospital-based prospective cohort study from Kerala, India. BMJ Paediatr Open 2021;5:e001195.
[Article] [PubMed] [PMC]56. Torres JP, Izquierdo G, Acuña M, Pavez D, Reyes F, Fritis A, et al. Multisystem inflammatory syndrome in children (MIS-C): Report of the clinical and epidemiological characteristics of cases in Santiago de Chile during the SARS-CoV-2 pandemic. Int J Infect Dis 2020;100:75–81.
[Article] [PubMed] [PMC]57. Valverde I, Singh Y, Sanchez-de-Toledo J, Theocharis P, Chikermane A, Di Filippo S, et al. Acute cardiovascular manifestations in 286 children with multisystem inflammatory syndrome associated with COVID-19 infection in Europe. Circulation 2021;143:21–32.
[Article] [PubMed]58. Abrams JY, Oster ME, Godfred-Cato SE, Bryant B, Datta SD, Campbell AP, et al. Factors linked to severe outcomes in multisystem inflammatory syndrome in children (MIS-C) in the USA: a retrospective surveillance study. Lancet Child Adolesc Health 2021;5:323–31.
[Article] [PubMed] [PMC]59. Atay G, Hasbal C, Türk M, Erdoğan S, Sözeri B. The role of therapeutic plasma exchange (TPE) in multisystem inflammatory syndrome in children (MIS-C). Children (Basel) 2021;8:498.
[Article] [PubMed] [PMC]60. Kıymet E, Böncüoğlu E, Şahinkaya Ş, Cem E, Çelebi MY, Düzgöl M, et al. A Comparative study of children with MIS-C between admitted to the pediatric intensive care unit and pediatric ward: a one-year retrospective study. J Trop Pediatr 2021;67:fmab104.
[PubMed]61. Emeksiz S, Özcan S, Perk O, Uyar E, Çelikel Acar B, Kibar Gül AE, et al. Therapeutic plasma exchange: a potential management strategy for critically ill MIS-C patients in the pediatric intensive care unit. Transfus Apher Sci 2021;60:103119.
[Article] [PubMed]62. Deep A, Upadhyay G, du Pré P, Lillie J, Pan D, Mudalige N, et al. Acute kidney injury in pediatric inflammatory multisystem syndrome temporally associated with severe acute respiratory syndrome coronavirus-2 pandemic: experience from PICUs across United Kingdom. Crit Care Med 2020;48:1809–18.
[Article] [PubMed]63. Fitzgerald JC, Weiss SL, Maude SL, Barrett DM, Lacey SF, Melenhorst JJ, et al. Cytokine release syndrome after chimeric antigen receptor T cell therapy for acute lymphoblastic leukemia. Crit Care Med 2017;45:e124–31.
[Article] [PubMed] [PMC]64. Simon DW, Halstead ES, Davila S, Kernan KF, Clark RSB, Storch G, et al. DNA Viremia is associated with hyperferritinemia in pediatric sepsis. J Pediatr 2019;213:82–7.e2.
[Article] [PubMed] [PMC]65. Cui Y, Xiong X, Ren Y, Wang F, Wang C, Zhang Y. CD163 as a valuable diagnostic and prognostic biomarker of sepsis-associated hemophagocytic lymphohistiocytosis in critically ill children. Pediatr Blood Cancer 2019;66:e27909.
[Article] [PubMed]66. Carcillo JA, Halstead ES, Hall MW, Nguyen TC, Reeder R, Aneja R, et al. Three hypothetical inflammation pathobiology phenotypes and pediatric sepsis-induced multiple organ failure outcome. Pediatr Crit Care Med 2017;18:513–23.
[Article] [PubMed] [PMC]67. Tonial CT, Garcia PCR, Schweitzer LC, Costa CAD, Bruno F, Fiori HH, et al. Cardiac dysfunction and ferritin as early markers of severity in pediatric sepsis. J Pediatr (Rio J) 2017;93:301–7.
[Article] [PubMed]68. Williams V, Menon N, Bhatia P, Biswal M, Sreedharanunni S, Rawat A, et al. Serum ferritin predicts neither organ dysfunction nor mortality in pediatric sepsis due to tropical infections. Front Pediatr 2020;8:607673.
[Article] [PubMed] [PMC]69. Tonial CT, Costa CAD, Andrades GRH, Crestani F, Bruno F, Piva JP, et al. Performance of prognostic markers in pediatric sepsis. J Pediatr (Rio J) 2021;97:287–94.
[Article] [PubMed] [PMC]70. Tonial CT, Costa CAD, Andrades GRH, Crestani F, Einloft PR, Bruno F, et al. Prediction of poor outcomes for septic children according to ferritin levels in a middle-income setting. Pediatr Crit Care Med 2020;21:e259–66.
[Article] [PubMed]71. Alzahrani FM, Sattar Shaikh S. Acinetobacter baumannii Infection in Transfusion Dependent Thalassemia Patients with Sepsis. Biomed Res Int 2017;2017:2351037.
[PubMed] [PMC]72. Ghosh S, Baranwal AK, Bhatia P, Nallasamy K. Suspecting hyperferritinemic sepsis in iron-deficient population: do we need a lower plasma ferritin threshold? Pediatr Crit Care Med 2018;19:e367–73.
[Article] [PubMed]73. Garcia PC, Longhi F, Branco RG, Piva JP, Lacks D, Tasker RC. Ferritin levels in children with severe sepsis and septic shock. Acta Paediatr 2007;96:1829–31.
[Article] [PubMed]74. Haiden N, Schwindt J, Cardona F, Berger A, Klebermass K, Wald M, et al. Effects of a combined therapy of erythropoietin, iron, folate, and vitamin B12 on the transfusion requirements of extremely low birth weight infants. Pediatrics 2006;118:2004–13.
[Article] [PubMed]75. Al-Ghananim RT, Nalbant D, Schmidt RL, Cress GA, Zimmerman MB, Widness JA. Reticulocyte hemoglobin content during the first month of life in critically Ill very low birth weight neonates differs from term infants, children, and adults. J Clin Lab Anal 2016;30:326–34.
[Article] [PubMed] [PMC]76. Widness JA, Serfass RE, Haiden N, Nelson SE, Lombard KA, Pollak A. Erythrocyte iron incorporation but not absorption is increased by intravenous iron administration in erythropoietin-treated premature infants. J Nutr 2006;136:1868–73.
[Article] [PubMed]77. Mäkelä E, Takala TI, Suominen P, Matomäki J, Salmi TT, Rajamäki A, et al. Hematological parameters in preterm infants from birth to 16 weeks of age with reference to iron balance. Clin Chem Lab Med 2008;46:551–7.
[PubMed]78. Ram Mohan G, Shashidhar A, Chandrakala BS, Nesargi S, Suman Rao PN. Umbilical cord milking in preterm neonates requiring resuscitation: a randomized controlled trial. Resuscitation 2018;130:88–91.
[Article] [PubMed]79. Amin SB, Scholer L, Srivastava M. Pre-discharge iron status and its determinants in premature infants. J Matern Fetal Neonatal Med 2012;25:2265–9.
[Article] [PubMed]80. Ochiai M, Kurata H, Inoue H, Tanaka K, Matsushita Y, Fujiyoshi J, et al. An elevation of serum ferritin level might increase clinical risk for the persistence of patent ductus arteriosus, sepsis and bronchopulmonary dysplasia in erythropoietin-treated very-low-birth-weight infants. Neonatology 2017;111:68–75.
[Article] [PubMed]81. Fernandes DM, Oliveira CR, Guerguis S, Eisenberg R, Choi J, Kim M, et al. Severe acute respiratory syndrome coronavirus 2 clinical syndromes and predictors of disease severity in hospitalized children and youth. J Pediatr 2021;230:23–31.e10.
[Article] [PubMed] [PMC]82. Hammad M, Shalaby L, Sidhom I, Sherief N, Abdo I, Soliman S, et al. Management and outcome of coronavirus disease 2019 (COVID-19) in pediatric cancer patients: a single centre experience from a developing country. Clin Lymphoma Myeloma Leuk 2021;21:e853–64.
[Article] [PubMed] [PMC]83. Balagurunathan M, Natarajan T, Karthikeyan J, Palanisamy V. Clinical spectrum and short-term outcomes of multisystem inflammatory syndrome in children in a south Indian hospital. Clin Exp Pediatr 2021;64:531–7.
[Article] [PubMed] [PMC]84. Acevedo L, Piñeres-Olave BE, Niño-Serna LF, Vega LM, Gomez IJA, Chacón S, et al. Mortality and clinical characteristics of multisystem inflammatory syndrome in children (MIS-C) associated with covid-19 in critically ill patients: an observational multicenter study (MISCO study). BMC Pediatr 2021;21:516.
[Article] [PubMed] [PMC]85. Carcillo JA, Sward K, Halstead ES, Telford R, Jimenez-Bacardi A, Shakoory B, et al. A systemic inflammation mortality risk assessment contingency table for severe sepsis. Pediatr Crit Care Med 2017;18:143–50.
[Article] [PubMed] [PMC]86. Kurokawa M, Nishiyama K, Koga Y, Eguchi K, Imai T, Oba U, et al. Hyperferritinemia and acute kidney injury in pediatric patients receiving allogeneic hematopoietic cell transplantation. Pediatr Nephrol 2020;35:1977–84.
[Article] [PubMed]87. Bennett TD, Hayward KN, Farris RW, Ringold S, Wallace CA, Brogan TV. Very high serum ferritin levels are associated with increased mortality and critical care in pediatric patients. Pediatr Crit Care Med 2011;12:e233–6.
[Article] [PubMed]88. Sachdeva R, Rice TB, Reisner B, Brundage N, Hulbert C, Kaminski A, et al. The impact of coronavirus disease 2019 pandemic on U.S. and Canadian PICUs. Pediatr Crit Care Med 2020;21:e643–50.
[Article] [PubMed] [PMC]89. Woodruff AG, Choong K. Long-term outcomes and the post-intensive care syndrome in critically Ill children: a North American perspective. Children (Basel) 2021;8:254.
[Article] [PubMed] [PMC]90. Ibiebele I, Algert CS, Bowen JR, Roberts CL. Pediatric admissions that include intensive care: a population-based study. BMC Health Serv Res 2018;18:264.
[Article] [PubMed] [PMC]91. Senna S, Ong C, Ng ZM, Lee JH. Long-term morbidities in children with critical illness: gaps and opportunities. Ann Acad Med Singap 2018;47:291–337.
[Article] [PubMed]92. Kaushal K, Kaur H, Sarma P, Bhattacharyya A, Sharma DJ, Prajapat M, et al. Serum ferritin as a predictive biomarker in COVID-19. A systematic review, meta-analysis and meta-regression analysis. J Crit Care 2022;67:172–81.
[Article] [PubMed] [PMC]93. Zhang X, Huang S, Xu H. Preliminary investigation of serum ferritin level and its reference interval in apparent healthy children population in provincial children’s hospital. J Lab Med 2022;46:121–4.
[Article]94. Oh HL, Lee JA, Kim DH, Lim JS. Reference values for serum ferritin and percentage of transferrin saturation in Korean children and adolescents. Blood Res 2018;53:18–24.
[Article] [PubMed] [PMC]95. Kang W, Barad A, Clark AG, Wang Y, Lin X, Gu Z, et al. Ethnic differences in iron status. Adv Nutr 2021;12:1838–53.
[Article] [PubMed] [PMC]96. Trafimow D, Wang T, Wang C. From a sampling precision perspective, skewness is a friend and not an enemy! Educ Psychol Meas 2019;79:129–50.
[Article] [PubMed] [PMC]97. Nahm FS. Receiver operating characteristic curve: overview and practical use for clinicians. Korean J Anesthesiol 2022;75:25–36.
[Article] [PubMed] [PMC]