All issues > Volume 67(1); 2024
X-linked hypophosphatemic rickets: from diagnosis to management
- Corresponding author: Hee Gyung Kang, MD, PhD. Department of Pediatrics, Seoul National University Children’s Hospital, 101 Daehak-ro, Jongno-gu, Seoul 03080, Korea Email: kanghg@snu.ac.kr, kanghg1@gmail.com
- Received December 18, 2022 Revised February 2, 2023 Accepted March 28, 2023
- Abstract
-
X-linked hypophosphatemia (XLH), the most common cause of hypophosphatemic rickets, affects one in every 20,000 people. Although conventional therapy for XLH was introduced approximately 4 decades ago, the temporary replacement of oral phosphate salts and activated vitamin D cannot completely control chronic hypophosphatemia, leaving patients with incomplete healing and residual skeletal deformity as well as at risk of endocrine abnormalities and adverse drug reactions. However, understanding the pathophysiology has led to the development of a targeted therapy, burosumab, a fibroblast growth factor-23 inhibitor that was recently approved in Korea for the treatment of XLH. This review provides insight into the diagnosis, evaluation, treatment, and recommended follow-up for a typical case of XLH and reviews its pathophysiology.
- Introduction
- Introduction
The kidney tubules reclaim the majority of nutrients filtered by glomerular filtration into the urinary space through diverse mechanisms. When the reabsorption of filtered phosphate is impaired by acquired tubular damage or genetic defects in sodium-phosphate cotransporters or their regulators, significant hypophosphatemia occurs. If this persists in children, rickets, a failure to mineralize growing bones, because phosphate is required with Ca to form hydroxyapatite and mineralize the bone [1]. The common cause of rickets is the nutritional deficiency of vitamin D or Ca intake, previously denoted calcipenic rickets, while phosphopenic or hypophosphatemic rickets is relatively rare and often has a genetic cause [2].X-linked hypophosphatemia (XLH; Mendelian Inheritance in Man #307800) is the most common cause of hypophosphatemic rickets, comprising 90% of familial cases and 70% of sporadic cases and affecting one of every 20,000 people in the general population [2,3]. It was first labeled vitamin D–resistant rickets in 1937, and its inheritance pattern of X-linked disorders was elucidated in 1958. Since female carriers of pathogenic variants are also affected, it is an X-linked–dominant disorder [4]. In 1972, the nature of XLH as an inborn error of phosphate transport was revealed, and the causative gene PHEX (phosphate-regulating gene with homologies to endopeptidases on the X chromosome) was discovered in 1995 [5,6]. Although conventional therapy for XLH was introduced approximately 4 decades ago, the temporary replacement of oral phosphate salts and activated vitamin D cannot completely control chronic hypophosphatemia, leaving patients with incomplete healing of rickets and residual skeletal deformity as well as at risk of endocrine abnormalities and adverse drug reactions [7]. However, understanding the pathophysiology has led to the development of a targeted therapy, burosumab, a fibroblast growth factor-23 (FGF23) inhibitor, which was recently approved in Korea for the treatment of XLH. This review provides insight into the diagnosis, evaluation, treatment, and recommended follow-up for a typical case of XLH and details its pathophysiology [8].
- Presentation and evaluation
- Presentation and evaluation
- 1. Clinical vignette part 1
- 1. Clinical vignette part 1
A 25-month-old girl visited the outpatient clinic with growth impairment. Her perinatal and family histories were unremarkable, and she was receiving vitamin D supplementation. Upon examination, her height was 77.6 cm (<3rd percentile), while her weight was 9.8 kg (5th to 10th percentile). Her laboratory test results were unremarkable (serum calcium [Ca], 9.8 mg/dL; serum creatinine [Cr], 0.39 mg/dL) except for an elevated alkaline phosphatase (ALP, 1,087 IU/L) and hypophosphatemia (serum phosphorus [P], 2.4 mg/dL). Radiography of the hand and knee revealed metaphyseal fraying and growth plate widening suggestive of rickets (Fig. 1). An additional workup for rickets showed a normal urine Ca/Cr ratio (0.04) with an elevated urine P level (75.2 mg/dL), low tubular reabsorption of phosphorus (TRP, 69%), and a low ratio of tubular maximum reabsorption of phosphorus to glomerular filtration rate (TmP/GFR, 1.65; reference range, 3.25–5.51). Serum 25-hydroxy (OH) vitamin D and parathyroid hormone (PTH) levels were within the normal ranges (45.47 ng/mL and 67.8 pg/mL, respectively), while the 1,25-dihydroxy vitamin D (1,25(OH)2D) levels was elevated (99.95 ng/mL). The genetic diagnosis of XLH was made by the identification of loss-of-function mutations of the PHEX gene.XLH usually presents as typical rickets, but its symptoms vary according to age at presentation and disease severity [9]. Short stature, bowing of the legs, difficulty walking from the deformity and weakness (waddling gait), and an unusual shape (dolichocephaly, frontal bossing) of the skull from craniosynostosis are common chief complaints [10-12]. The family history may be significant in X-linked–dominant patterns for disproportionate short stature, leg deformities, dental abscesses, periodontal disease, and hearing loss along with osteoarthritis, enthesopathy, and (pseudo) fractures [2]. Upon examination, typical findings of rickets, namely widening of the wrists and metaphysis and valgus or varus deformities of the legs, are noted. Often, the children of an affected female patient visit the clinic and are diagnosed through laboratory tests before the disease develops. Laboratory characteristics of XLH include hypophosphatemia, normocalcemia, hyperphosphaturia (low TRP and TmP/GFR), and inappropriately normal 1,25(OH)2D levels [13,14]. While normal PTH is a typical finding of XLH, if the patient is taking supplemental vitamin D, PTH and 1,25(OH)2D might be mildly elevated as shown in the vignette.
- Pathophysiology
- Pathophysiology
To understand the pathophysiology of XLH, it is necessary to understand the regulatory mechanisms of Ca and phosphate (Fig. 2). When serum P is decreased, its intestinal absorption and tubular reabsorption are increased through the sodium-coupled phosphate cotransporters NaPi2b (intestine), NaPi2a, and NaPi2c (kidney), which are upregulated by increased 1,25(OH)2D production. With hyperphosphatemia, the phosphaturic hormone FGF23 is activated. It then lowers serum P by decreasing renal tubular reabsorption of phosphate through the downregulation of NaPi2a and NaPi2c and suppresses the activity of renal 25(OH)D3 1α-hydroxylase to reduce the production of 1,25(OH)2D [15-19]. In the case of hypocalcemia, PTH is upregulated to increase serum Ca by mobilizing it from the bone, increasing the production of 1,25(OH)2D to absorb/reabsorb more Ca from the intestine and kidney, and downregulating kidney tubular reabsorption of P [20]. In XLH, the FGF23 level is increased regardless of the serum P level, causing phosphaturia and decreased or inappropriately normal 1,25(OH)2D levels, the main pathogenic mechanism in XLH (Fig. 2) [21]. However, which is the mechanism by which FGF23 is increased in XLH has not yet been elucidated [22-24]. Additionally, other regulators of bone mineralization, such as osteopontin and acid serine aspartate–rich, MEPE-associated protein peptides, also increase in XLH [24,25]. On the other hand, such an increase in FGF23 leading to phosphaturia is not unique to XLH. Still, other genetic conditions affecting FGF23 or its regulators have a similar pathophysiology (Table 1, Fig. 3). In recent studies, the detection rate of PHEX mutations was reportedly 33.0%–83.3% when patients with phosphopenic rickets were screened by targeted next-generation sequencing [26-28]. Therefore, a confirmational diagnosis of XLH is usually obtained only after a genetic diagnosis is made.
- Differential diagnosis
- Differential diagnosis
The typical clinical picture of hypophosphatemic rickets occurs in conditions other than XLH such as dietary phosphate deficiency, including very low birth weight exclusively breastfed infants, infants fed elemental or hypoallergenic formulas, and patients receiving parenteral nutrition as well as impaired phosphate bioavailability due to overuse of P binders and gastrointestinal disorders [2,28-30]. Other conditions, such as primary renal tubulopathies, may resemble XLH, including hereditary hypophosphatemic rickets with hypercalciuria, Dent disease 1, cystinosis, other hereditary forms of Fanconi syndrome, and iatrogenic proximal tubulopathy [30].
- Treatment
- Treatment
- 1. Clinical vignette part 2
- 1. Clinical vignette part 2
The patient was prescribed alfacalcidol 0.25 µg once a day and potassium phosphate/sodium phosphate 125 mg (Phospha 250 Neutral 0.5 tablet) 4 times a day. She will be followed up monthly until her ALP level normalizes, quarterly until 5 years of age, every 3–6 months until puberty, and more frequently during puberty according to the guidelines [31]. Growth, neurological signs, and metabolic control will be monitored for normal ALP, PTH, and normocalciuria. Blood pressure and dental examinations twice a year, renal ultrasonography and an orthopedic examination once a year, and yearly hearing tests from 8 years of age will also be performed. Once available, burosumab, an anti-FGF23, will be prescribed until her growth is complete.XLH was historically managed with oral phosphate supplementation and activated vitamin D (calcitriol or alfacalcidol) to offset its renal loss. The recommended doses of each medication are listed in Table 2 [31-33]. Once provided with phosphate and active vitamin D, rickets symptoms are usually ameliorated and patients grow better and complain of less bone pain [34-36]. However, the efficacy of such management is often insufficient, as the skeletal deformity progresses and osteotomy becomes necessary in many cases [37,38]. In addition, a large amount of phosphate is necessary to normalize serum Plevels, excessive amounts of which inhibit intestinal Ca absorption, leading to secondary hyperparathyroidism and aggravating bone resorption and phosphaturia [39]. However, an excessive calcitriol dosage may cause hypercalciuria and nephrocalcinosis. Nephrocalcinosis is a common complication of XLH, not from the disease itself but from the replacement therapy [40,41]. Therefore, clinicians need to well titrate and balance the dosage of phosphate supplementation and active vitamin D to maintain normal PTH levels and normocalciuria.The target of XLH management is the recovery from rickets with the normalization of serum ALP levels but not the normalization of serum P levels. Another problem with classical treatment is poor compliance owing to gastrointestinal problems caused by phosphate supplementation.- 2. Anti-FGF23 treatment
- 2. Anti-FGF23 treatment
As an increase in FGF23 is the main pathogenic mechanism of XLH, the inhibition of FGF23 may be the ideal treatment approach. Burosumab, a fully humanized monoclonal immunoglobulin G1 antibody that neutralizes FGF23, was developed in the early 2000s and showed efficacy and safety in a series of clinical trials in adults and children [42-48]. This targeted medicine increases serum P levels and improves physical function.In an open-label, phase 2 trial of XLH children, 52 patients aged 5–12 years were randomly assigned to receive burosumab every 2 weeks or every 4 weeks for 64 weeks. Every 2 weeks dosing improved TRP with more stable serum P levels than every 4 weeks dosing and resulted in substantial healing of rickets in nearly all the children with severe disease. These results indicate that the administration of burosumab every 2 weeks is an appropriate regimen for children with XLH [44]. In an active-controlled, open-label, phase 3 trial of XLH children, 61 patients aged 1–12 years of age were randomly assigned to receive burosumab subcutaneously every 2 weeks or conventional therapy for 40 weeks. Rickets severity and height z-score improved significantly more in the burosumab versus conventional therapy group [46]. In another open-label phase 2 trial of XLH children aged 1–4 years of age, 13 patients received burosumab every 2 weeks for 64 weeks. In this study, burosumab increased serum P levels, improved the rickets, and prevented an early decline in height z-score [48].Additional benefits of this targeted therapy include elimination of the burden of frequent medication dosing and the side effects of conventional medications of gastrointestinal discomfort, secondary hyperparathyroidism, hypercalciuria, and nephrocalcinosis. In clinical trials of XLH children, most patients who received burosumab experienced an adverse effect, but most were mild or moderate in severity with the most common being injection site reactions, hypersensitivity, headache, cough, vomiting and pyrexia [44,46,48]. As a result, burosumab was approved for the treatment of XLH and tumor-induced osteomalacia in patients older than 1 year with radiographic evidence of bone disease in the United States, Europe, and Japan in 2018, and in 2020 in Korea. It is expected to be available in Korea in early 2023 [8].The dosage of burosumab is usually titrated to achieve a lower age-related normal range of serum P levels [32]. For growing children, its starting dosage is 0.8 mg/kg every 2 weeks subcutaneously (not to exceed 90 mg; after the completion of growth, 1 mg/kg every 4 weeks subcutaneously) and increased by 0.4 mg/kg (up to 2.0 mg/kg) every 4 weeks to raise fasting serum P levels within the lower end of the normal reference range for age. Burosumab should be withheld if the fasting serum P level at 7–11 days postinjection exceeds the upper range of normal and can be restarted at approximately half of the previous dose when the serum P concentration is below the normal range [32]. Assessments of TmP/GFR are recommended to confirm improvement in renal phosphate wasting [31].
- Follow-up evaluation
- Follow-up evaluation
Recommended follow-up intervals and evaluations are listed in Table 3. In addition to disease activity and growth, treatment-associated side effects such as hypercalciuria, nephrocalcinosis, nephrolithiasis, abnormal PTH levels (high or low) in patients receiving conventional treatment, and hyperphosphatemia or hypervitaminosis D (1,25(OH)2D) in patients receiving burosumab treatment can occur [31]. Since XLH is accompanied by complications encompassing orthopedics, neurosurgery, dentistry, and otolaryngology, careful evaluations for such conditions are also needed. Notably, the majority of adult and children with XLH experience bone, joint, and/or muscle pain. Craniosynostosis is observed in about one-third of patients with hypophosphatemia; thus, strict monitoring of head circumference and skull shape during the early years of life is essential. The possibility of increased intracranial pressure, headache, and neck pain from craniosynostosis, a type 1 Chiari malformation, or syringomyelia requires consideration [4,11,12,31,49]. Also, since approximately two-thirds of patients with XLH suffer from dental and periodontal lesions, regular dental examinations to screen for delayed dentition and tooth abscesses are necessary. Hearing problems are rarely seen in children with XLH, but various degrees of hearing loss can occur in adults with XLH, so evaluations for hearing loss starting at 8 years of age are recommended [4,50-52].Radiographic evidence of bone diseases can be quantitatively assessed by the Rickets Severity Score (RSS) using radiographs of the wrists and knees (Table 4, Fig. 4) [50,53]. The RSS is a validated measure of rickets severity ranging from 0 (no rickets) to 10 (severe rickets) based on the degree of metaphyseal fraying and concavity and the proportion of affected growth plate affected (https://www.rsstrainingtool.net/).
- Prognosis
- Prognosis
Once an individual fully matures, their requirement for Ca and phosphate decreases with the spontaneous amelioration of hypophosphatemia, albeit with incomplete resolution. The final height of the XLH patient depends on interventional timing and mode [25,54]. The efficacy of growth hormone treatment is uncertain, especially among those with less optimal metabolic control [54-58]. Residual lower-leg deformities are often debilitating, and about half of affected individuals require surgical correction consisting of corrective osteotomies for those who attain their adult height and epiphysiodesis for those who are still growing [31,37,38,49]. Optimal metabolic control is a prerequisite for surgery. In addition to deformities, osteophytes, enthesopathies (ossification of the tendon), osteoarthritis, (pseudo)fractures, and spinal stenosis may occur, resulting in pain and/or immobility [7,31,59,60]. Supplemental phosphate and active vitamin D may relieve the symptoms [61,62]. Dental care is important for patients with XLH because dental abscesses, poorly mineralized dentin, and periodontitis are common. Although conventional treatment with oral phosphate and active vitamin D may ameliorate this problem, the efficacy of burosumab in this area remains unclear [4,61].
- Conclusion
- Conclusion
Although rare, XLH is a clinically significant disease whose delayed management may result in short stature and debilitating deformity. Especially since a targeted therapy based on its pathophysiology is now available, the suspicion and early diagnosis of XLH in every case of short stature and hyperphosphaturic hypophosphatemia is necessary.
- Footnotes
-
Conflicts of interest No potential conflict of interest relevant to this article was reported.
Funding This work was supported by the National Research Foundation of Korea (NRF) grant funded by the Korean government (MSIT) (No. 2020R1A2C1100974).
-
Fig. 2.
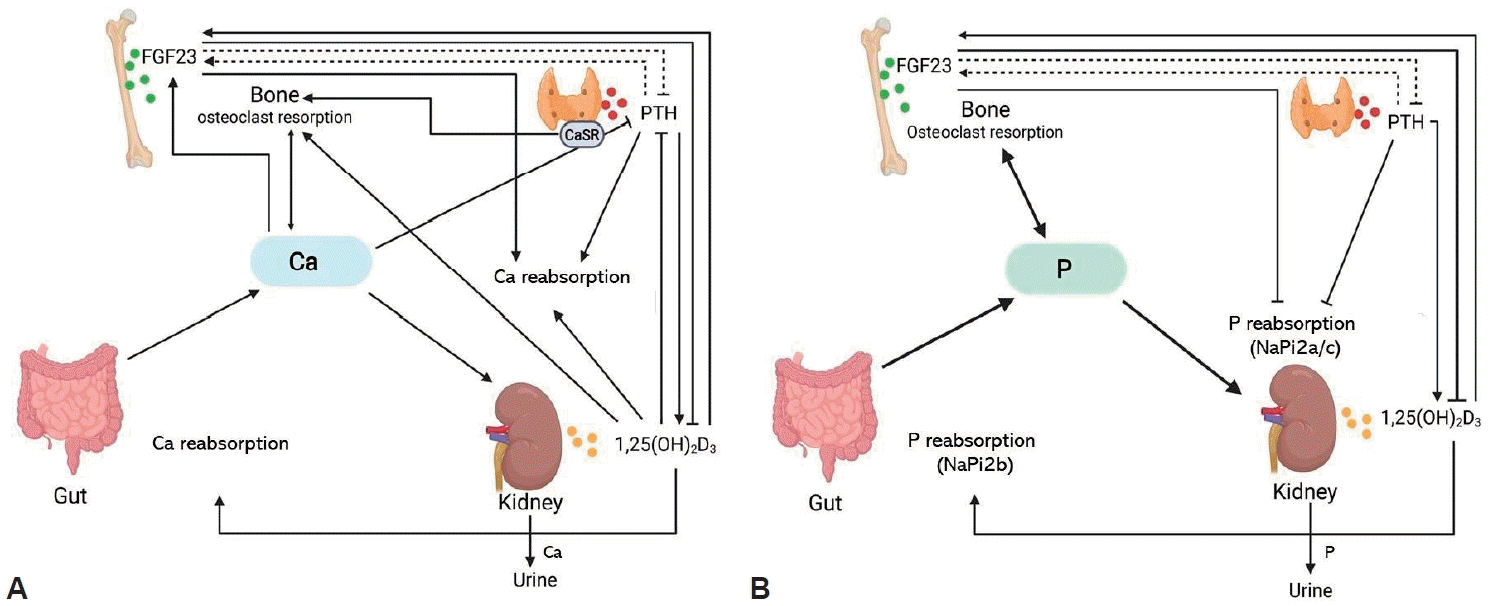
Fig. 3.

Fig. 4.
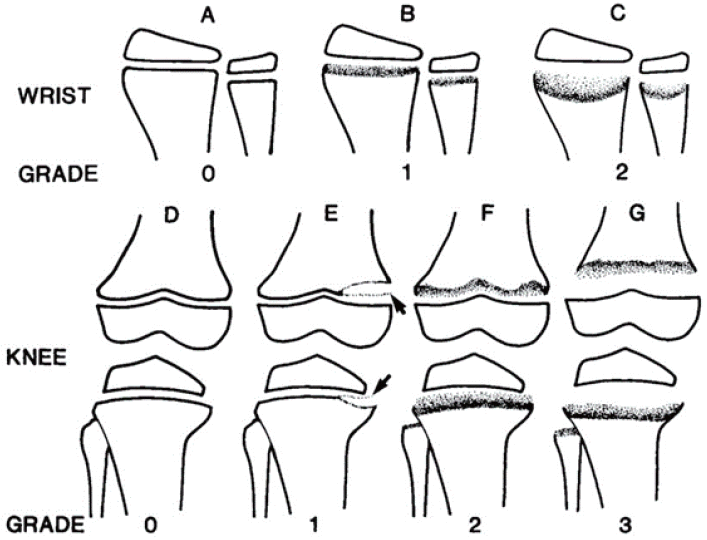
Table 1.
Disorder (abbreviation; OMIM#) | Gene (location) | Ca | P | ALP | UCa/Crea | UP/Crea | TmP/GFR | FGF23 | PTH | 25(OH)Da) | 1,25(OH)2D |
---|---|---|---|---|---|---|---|---|---|---|---|
X-linked hypophosphatemia (XLH; OMIM#307800) | PHEX (Xp22.1) | N | ↓ | ↑, ↑↑ | ↓ | ↑ | ↓ | ↑, N | N,↑c) | N | Nb) |
AD hypophosphatemic rickets (ADHR; OMIM#193100) | FGF23 (12p13.3) | N | ↓ | ↑, ↑↑ | ↓ | ↑ | ↓ | ↑, N | N,↑c) | N | Nb) |
AR hypophosphatemic rickets 1 (ADHR1; OMIM#241520) | DMP1 (4q22.1) | N | ↓ | ↑, ↑↑ | ↓ | ↑ | ↓ | ↑, N | N,↑c) | N | Nb) |
AR hypophosphatemic rickets 2 (ADHR2; OMIM#613312) | ENPP1 (6q23.2) | N | ↓ | ↑, ↑↑ | ↓ | ↑ | ↓ | ↑, N | N,↑c) | N | Nb) |
Raine syndrome-associated (ARHR3; OMIM#259775) | FAM20C (7q22.3) | N | ↓ | ↑, ↑↑ | ? | ↑ | ↓ | ↑, N | N,↑c) | N | Nb) |
Fibrous dysplasia (FD; OMIM#174800) | GNAS (20q13.3) | N, ↓ | ↓ | ↑, ↑↑ | ↓ | ↑ | ↓ | N,↑ | N,↑c) | N | Nb) |
Tumor-induced osteomalacia (TIO) | NA | N, ↓ | ↓ | ↑, ↑↑ | ↓ | ↑ | ↓ | N,↑ | N,↑c) | N | Nb) |
Cutaneous skeletal hypophosphatemia syndrome (SFM; OMIM#163200) | NRAS (1p13.2) | N, ↓ | ↓ | ↑, ↑↑ | ↓ | ↑ | ↓ | N,↑ | N,↑c) | N | Nb) |
HRAS (11p15.5) | |||||||||||
KRAS (12p12.1) | |||||||||||
Osteoglophonic dysplasia (OGD; OMIM#612089) | FGFR1 (8p11.23) | N | ↓ | ↑, N | N | ↑ | ↓ | N | N,↑c) | N | Nb) |
Hypophosphatemic rickets and hyperparathyroidism (OMIM#612089) | KLOTHO (13q13.1) | N | ↓ | ↑, ↑↑ | ↓ | ↑ | ↓ | ↑ | ↑↑ | N | Nb) |
Rickets and/or osteomalacia with high PTH levels (calcipenic rickets) | |||||||||||
Nutritional rickets or Vitamin D-dependent rickets | N, ↓ | N, ↓ | ↑, ↑↑ | ↓ | Varies | ↓ | N,↓ | ↑↑↑ | ↓↓, N | Varies |
Ca, calcium; P, phosphorous; ALP, alkaline phosphatase; UCa/Crea, urinary calcium to creatinine ratio; UP/Crea, urinary phosphate to creatinine ratio; TmP/GFR, maximum rate of renal tubular reabsorption of phosphate normalized to the glomerular filtration rate; FGF23, fibroblast growth factor-23; NA, not applicable; PTH, parathyroid hormone; 25(OH)D, calcidiol; 1,25(OH)2D, 1,25-dihydroxyvitamin; N, normal; ↑, elevated; ↑↑, very elevated; ↑ (↑↑), may range widely.
c) PTH may be moderately elevated; Modified from Haffner D, et al. Pediatr Nephrol 2022;37:2013-36. [2]
Table 2.
Drug | Dosage |
---|---|
Phosphatea) given in 4–6 doses | Elemental P 20–60 mg/kg/day (0.7–2.0 mmol/kg/day) |
Maximum 80 mg/kg | |
Calcitriolb) given in 1–2 doses | 20–30 ng/kg/day, alternatively, 0.5 μgc) (age > 12 months) |
Alphacalcidiolb) (ng/kg) given once daily | 30–50 ng/kg/day, alternatively, 1 μgc) (age > 12 months) |
Vitamn D2 or D3 | In case of vitamin D deficiency |
Age-appropriate daily calcium intake | 500-mg Ca in children >12 months |
a) Based on elemental phosphorus; infants and young children usually require more frequent phosphate administrations than older children and adolescents.
b) Phosphate should always be given in combination with either calcitriol or alphacalcidiol or alphacalcidiol.
c) Starting dose; other forms of fibroblast-growth factor 23-associated hypophosphatemic rickets are usually treated with similar doses, but evidence-based recommendations or consensus statements are lacking.
Modified from Haffner et al. Pediatr Nephrol 2022;37:2289-302. [31]
Table 3.
Examination | 0–5 Years | 5 Years-start of puberty (9–12 years) | Puberty |
---|---|---|---|
Frequency of visits | Monthly-3 monthly | 3–6 Months | 3 Months |
Height, weight, IMD, ICDa) | V | V | V |
Head circumference and skull shape | V | NA | NA |
Presence of rickets, pain, stiffness, fatigue, muscle weakness, gait pattern | V | V | V |
Neurological examinationb) | V | V | V |
Orthopedic examination | Once a year in the presence of significant leg bowing | ||
Dental examination | Twice-yearly after tooth eruption | Twice-yearly | Twice-yearly |
Hearing test | Not feasible | From 8 years: hearing evaluation if symptoms of hearing difficulties | |
Serum levels of ALP, Ca, Pi, PTH, Crea; eGFR | V | V | V |
25(OH) vitamin D levels | After 3–4 weeks in nutritional rickets, yearly in other rickets forms | ||
1,25(OH)2 vitamin D levels | Every 3–6 months in patients on burosumab treatment, those on active vitamin D | ||
UCa/Crea TmP/GFR | Every 3–6 months in patients on active vitamin D or burosumab treatment. Initially, at every visit in patients on burosumab treatment | ||
Blood pressure | Twice yearly | ||
Kidney ultrasonography | Every 1–2 years on phosphate, active vitamin D or burosumab treatment | ||
Left wrist and/or lower limbs radiographs | If leg bowing does not improve upon treatment | In adolescents with persistent lower limb deformities when they are transitioning to adult care | |
If surgery is indicated | |||
Dental orthopantomogram | Not feasible | Based on clinical needs | |
Funduscopy and brain MRI | If aberrant shape of skull, headaches, or neurological symptoms | If recurrent headaches, declining school/cognitive performances, or neurological symptoms |
IMD, intermalleolar distance; ICD, intercondylar distance; NA, not applicable; ALP, alkaline phosphatase; Ca, calcium; Pi, inorganic phosphate; PTH, parathyroid hormone; Crea, creatinine; eGFR, estimated glomerular filtration rate; 25(OH)D, calcidiol; 1,25(OH)2D, 1,25-dihydroxyvitamin; UCa/Crea, urinary calcium to creatinine ratio; TmP/GFR, maximum rate of tubular reabsorption of phosphate per glomerular filtration rate; MRI, magnetic resonance imaging.
TmP/GFR is calculated by entering the fasting urine and plasma concentrations, in the same concentration units, into the following equation: TmP/GFR=Pp–(Up/Ucr)×Pcr. TmP/GFR, ratio of tubular maximum reabsorption of phosphorus to glomerular filtration rate; Pp, plasma phosphate; Up, urine phosphate; UCr, urine creatinine; PCr, plasma creatinine
b) Consequences of craniosynostosis and spinal stenosis. Modified from Haffner et al. Pediatr Nephrol 2022;37:2289-302. [31]
Table 4.
Total possible score is 10, with 4 for the wrist, and 6 for the knee.
Adapted from Thacher et al. J Trop Pediatr 2000;46:132-9, [53] with permission from Oxford University Press.
- References
- 1. Ackah SA, Imel EA. Approach to hypophosphatemic rickets. J Clin Endocrinol Metab 2022;108:209–20.
[Article] [PubMed] [PMC]2. Haffner D, Leifheit-Nestler M, Grund A, Schnabel D. Rickets guidance: part I-diagnostic workup. Pediatr Nephrol 2022;37:2013–36.
[Article] [PubMed] [PMC]3. Ruppe MD. X-linked hypophosphatemia. 2012 Feb 9 [].In: Adam MP, Mirzaa GM, Pagon RA, Wallace SE, Bean LJH, Gripp KW, et al., editors. GeneReviews® [Internet]. Seattle (WA): University of Washington, Seattle; 1993–2023.4. Baroncelli GI, Mora S. X-linked hypophosphatemic rickets: multisystemic disorder in children requiring multidisciplinary management. Front Endocrinol (Lausanne) 2021;12:688309.
[Article] [PubMed] [PMC]5. The HYP Consortium. A gene (PEX) with homologies to endopeptidases is mutated in patients with X-linked hypophosphatemic rickets. Nat Genet 1995;11:130–6.
[Article] [PubMed]6. Beck L, Soumounou Y, Martel J, Krishnamurthy G, Gauthier C, Goodyer CG, et al. Pex/PEX tissue distribution and evidence for a deletion in the 3' region of the PEX gene in X-linked hypophosphatemic mice. J Clin Invest 1997;99:1200–9.
[Article] [PubMed] [PMC]7. Skrinar A, Dvorak-Ewell M, Evins A, Macica C, Linglart A, Imel EA, et al. The lifelong impact of X-linked hypophosphatemia: results from a burden of disease survey. J Endocr Soc 2019;3:1321–34.
[Article] [PubMed] [PMC]8. Emma F, Haffner D. FGF23 blockade coming to clinical practice. Kidney Int 2018;94:846–8.
[Article] [PubMed]9. Veilleux LN, Cheung M, Amor M, Rauch F. Abnormalities in muscle density and muscle function in hypophosphatemic rickets. J Clin Endocrinol Metab 2012;97:E1492–8.
[Article] [PubMed]10. Collins MT, Marcucci G, Anders HJ, Beltrami G, Cauley JA, Ebeling PR, et al. Skeletal and extraskeletal disorders of biomineralization. Nat Rev Endocrinol 2022;18:473-89. Nat Rev Endocrinol 2022;18:473. –89. Erratum in: Nat Rev Endocrinol 2023;19:62.
[Article] [PubMed]11. Rothenbuhler A, Fadel N, Debza Y, Bacchetta J, Diallo MT, Adamsbaum C, et al. High incidence of cranial synostosis and chiari I malformation in children with X-linked hypophosphatemic rickets (XLHR). J Bone Miner Res 2019;34:490–6.
[Article] [PubMed] [PMC]12. Glorieux FH, Bonewald LF, Harvey NC, van der Meulen MCH. Potential influences on optimizing long-term musculoskeletal health in children and adolescents with X-linked hypophosphatemia (XLH). Orphanet J Rare Dis 2022;17:30.
[Article] [PubMed] [PMC]13. Gohil A, Imel EA. FGF23 and associated disorders of phosphate wasting. Pediatr Endocrinol Rev 2019;17:17–34.
[PubMed] [PMC]14. Florenzano P, Cipriani C, Roszko KL, Fukumoto S, Collins MT, Minisola S, et al. Approach to patientswith hypophosphataemia. Lancet Diabetes Endocrinol 2020;8:163–74.
[Article] [PubMed]15. Kritmetapak K, Kumar R. Phosphatonins: from discovery to therapeutics. Endocr Pract 2023;29:69–79.
[Article] [PubMed]16. Michigami T. Roles of osteocytes in phosphate metabolism. Front Endocrinol (Lausanne) 2022;13:967774.
[Article] [PubMed] [PMC]17. Nakatani S, Nakatani A, Mori K, Emoto M, Inaba M, Razzaque MS. Fibroblast growth factor 23 as regulator of vitamin D metabolism. Adv Exp Med Biol 2022;1362:47–54.
[Article] [PubMed]18. Shimada T, Hasegawa H, Yamazaki Y, Muto T, Hino R, Takeuchi Y, et al. FGF-23 is a potent regulator of vitamin D metabolism and phosphate homeostasis. J Bone Miner Res 2004;19:429–35.
[Article] [PubMed]19. Balani S, Perwad F. Fibroblast growth factor 23 and phosphate homeostasis. Curr Opin Nephrol Hypertens 2019;28:465–73.
[Article] [PubMed]20. Christakos S, Veldurthy V, Patel N, Wei R. Intestinal regulation of calcium: vitamin D and bone physiology. Adv Exp Med Biol 2017;1033:3–12.
[Article] [PubMed]21. Liu S, Zhou J, Tang W, Jiang X, Rowe DW, Quarles LD. Pathogenic role of Fgf23 in Hyp mice. Am J Physiol Endocrinol Metab 2006;291:E38–49.
[Article] [PubMed]22. Saito H, Maeda A, Ohtomo S, Hirata M, Kusano K, Kato S, et al. Circulating FGF-23 is regulated by 1alpha,25-dihydroxyvitamin D3 and phosphorus in vivo. J Biol Chem 2005;280:2543–9.
[PubMed]23. Liu S, Guo R, Simpson LG, Xiao ZS, Burnham CE, Quarles LD. Regulation of fibroblastic growth factor 23 expression but not degradation by PHEX. J Biol Chem 2003;278:37419–26.
[Article] [PubMed]24. Barros NM, Hoac B, Neves RL, Addison WN, Assis DM, Murshed M, et al. Proteolytic processing of osteopontin by PHEX and accumulation of osteopontin fragments in hyp mouse bone, the murine model of X-linked hypophosphatemia. J Bone Miner Res 2013;28:688–99.
[Article] [PubMed]25. Beck-Nielsen SS, Mughal Z, Haffner D, Nilsson O, Levtchenko E, Ariceta G, et al. FGF23 and its role in X-linked hypophosphatemia-related morbidity. Orphanet J Rare Dis 2019;14:58.
[Article] [PubMed] [PMC]26. Marik B, Bagga A, Sinha A, Khandelwal P, Hari P, Sharma A. Genetic and clinical profile of patients with hypophosphatemic rickets. Eur J Med Genet 2022;65:104540.
[Article] [PubMed]27. Turan I, Erdem S, Kotan LD, Ozdemir Dilek S, Tastan M, Gurbuz F, et al. Experience with the targeted next-generation sequencing in the diagnosis of hereditary hypophosphatemic rickets. J Pediatr Endocrinol Metab 2021;34:639–48.
[Article] [PubMed]28. Beck-Nielsen SS, Brixen K, Gram J, Brusgaard K. Mutational analysis of PHEX, FGF23, DMP1, SLC34A3 and CLCN5 in patients with hypophosphatemic rickets. J Hum Genet 2012;57:453–58.
[Article] [PubMed]29. Imel EA, Econs MJ. Approach to the hypophosphatemic patient. J Clin Endocrinol Metab 2012;97:696–706.
[Article] [PubMed] [PMC]30. Penido MG, Alon US. Hypophosphatemic rickets due to perturbations in renal tubular function. Pediatr Nephrol 2014;29:361–73.
[Article] [PubMed]31. Haffner D, Emma F, Eastwood DM, Duplan MB, Bacchetta J, Schnabel D, et al. Clinical practice recommendations for the diagnosis and management of X-linked hypophosphataemia. Nat Rev Nephrol 2019;15:435–55.
[Article] [PubMed] [PMC]32. Haffner D, Leifheit-Nestler M, Grund A, Schnabel D. Rickets guidance: part II-management. Pediatr Nephrol 2022;37:2289–302.
[Article] [PubMed] [PMC]33. Trombetti A, Al-Daghri N, Brandi ML, Cannata-Andía JB, Cavalier E, Chandran M, et al. Interdisciplinary management of FGF23-related phosphate wasting syndromes: a Consensus Statement on the evaluation, diagnosis and care of patients with X-linked hypophosphataemia. Nat Rev Endocrinol 2022;18:366–84.
[Article] [PubMed]34. Alikasifoglu A, Unsal Y, Gonc EN, Ozon ZA, Kandemir N, Alikasifoglu M. Long-term effect of conventional phosphate and calcitriol treatment on metabolic recovery and catch-up growth in children with PHEX mutation. J Pediatr Endocrinol Metab 2021;34:1573–84.
[Article] [PubMed]35. Cagnoli M, Richter R, Böhm P, Knye K, Empting S, Mohnike K. Spontaneous growth and effect of early therapy with calcitriol and phosphate in X-linked hypophosphatemic rickets. Pediatr Endocrinol Rev 2017;15:119–22.
[PubMed]36. Mäkitie O, Doria A, Kooh SW, Cole WG, Daneman A, Sochett E. Early treatment improves growth and biochemical and radiographic outcome in X-linked hypophosphatemic rickets. J Clin Endocrinol Metab 2003;88:3591–7.
[Article] [PubMed]37. Horn A, Wright J, Bockenhauer D, Van’t Hoff W, Eastwood DM. The orthopaedic management of lower limb deformity in hypophosphataemic rickets. J Child Orthop 2017;11:298–305.
[Article] [PubMed] [PMC]38. Paludan CG, Thomsen KKV, Rahbek O, Kold S. Complications of orthopedic treatment in patients diagnosed with X-linked hypophosphatemic rickets. J Pediatr Endocrinol Metab 2022;35:1003–9.
[Article] [PubMed]39. Lecoq AL, Chaumet-Riffaud P, Blanchard A, Dupeux M, Rothenbuhler A, Lambert B, et al. Hyperparathyroidism in patients with X-linked hypophosphatemia. J Bone Miner Res 2020;35:1263–73.
[Article] [PubMed]40. Colares Neto GP, Ide Yamauchi F, Hueb Baroni R, de Andrade Bianchi M, Cavalanti Gomes A, Chammas MC, et al. Nephrocalcinosis and nephrolithiasis in X-linked hypophosphatemic rickets: diagnostic imaging and risk factors. J Endocr Soc 2019;3:1053–61.
[Article] [PubMed] [PMC]41. Harada D, Ueyama K, Oriyama K, Ishiura Y, Kashiwagi H, Yamada H, et al. Switching from conventional therapy to burosumab injection has the potential to prevent nephrocalcinosis in patients with X-linked hypophosphatemic rickets. J Pediatr Endocrinol Metab 2021;34:791–98.
[Article] [PubMed]42. Carpenter TO, Imel EA, Ruppe MD, Weber TJ, Klausner MA, Wooddell MM, et al. Randomized trial of the anti-FGF23 antibody KRN23 in X-linked hypophosphatemia. J Clin Invest 2014;124:1587–97.
[Article] [PubMed] [PMC]43. Imel EA, Zhang X, Ruppe MD, Weber TJ, Klausner MA, Ito T, et al. Prolonged correction of serum phosphorus in adults with X-linked hypophosphatemia using monthly doses of KRN23. J Clin Endocrinol Metab 2015;100:2565. –73. Erratum in: J Clin Endocrinol Metab 2017;102:336.
[Article] [PubMed] [PMC]44. Carpenter TO, Whyte MP, Imel EA, Boot AM, Högler W, Linglart A, et al. Burosumab therapy in children with X-linked hypophosphatemia. N Engl J Med 2018;378:1987–98.
[Article] [PubMed]45. Insogna KL, Briot K, Imel EA, Kamenický P, Ruppe MD, Portale AA, et al. A randomized, double-blind, placebo-controlled, phase 3 trial evaluating the efficacy of burosumab, an anti-FGF23 antibody, in adults with X-linked hypophosphatemia: week 24 primary analysis. J Bone Miner Res 2018;33:1383–93.
[Article] [PubMed]46. Glorieux FH, Nilsson O, et al. Burosumab versus conventional therapy in children with X-linked hypophosphataemia: a randomised, activecontrolled, open-label, phase 3 trial. Lancet 2019;393:2416. –27. Erratum in: Lancet 2019;394:120.
[Article] [PubMed] [PMC]47. Insogna KL, Rauch F, Kamenický P, Ito N, Kubota T, Nakamura A, et al. Burosumab improved histomorphometric measures of osteomalacia in adults with X-linked hypophosphatemia: a phase 3, single-arm, international trial. J Bone Miner Res 2019;34:2183–91.
[Article] [PubMed] [PMC]48. Whyte MP, Carpenter TO, Gottesman GS, Mao M, Skrinar A, San Martin J, et al. Efficacy and safety of burosumab in children aged 1-4 years with X-linked hypophosphataemia: a multicentre, open-label, phase 2 trial. Lancet Diabetes Endocrinol 2019;7:189–99.
[Article] [PubMed]49. Rocco FD, Rothenbuhler A, Adamsbaum C, Bacchetta J, Pejin Z, Finidori G, et al. Orthopedic and neurosurgical care of X-linked hypophosphatemia. Arch Pediatr 2021;28:599–605.
[Article] [PubMed]50. Biosse Duplan M, Coyac BR, Bardet C, Zadikian C, Rothenbuhler A, Kamenicky P, et al. Phosphate and vitamin D prevent periodontitis in X-linked hypophosphatemia. J Dent Res 2017;96:388–95.
[Article] [PubMed]51. Hanisch M, Bohner L, Sabandal MMI, Kleinheinz J, Jung S. Oral symptoms and oral health-related quality of life of individuals with x-linked hypophosphatemia. Head Face Med 2019;15:8.
[Article] [PubMed] [PMC]52. Nakamura Y, Takagi M, Takeda R, Miyai K, Hasegawa Y. Hypertension is a characteristic complication of X-linked hypophosphatemia. Endocr J 2017;64:283–9.
[Article] [PubMed]53. Thacher TD, Fischer PR, Pettifor JM, Lawson JO, Manaster BJ, Reading JC. Radiographic scoring method for the assessment of the severity of nutritional rickets. J Trop Pediatr 2000;46:132–9.
[Article] [PubMed]54. André J, Zhukouskaya VV, Lambert AS, Salles JP, Mignot B, Bardet C, et al. Growth hormone treatment improves final height in children with X-linked hypophosphatemia. Orphanet J Rare Dis 2022;17:444.
[PubMed] [PMC]55. Meyerhoff N, Haffner D, Staude H, Wühl E, Marx M, Beetz R, et al. Effects of growth hormone treatment on adult height in severely short children with X-linked hypophosphatemic rickets. Pediatr Nephrol 2018;33:447–56.
[Article] [PubMed]56. Drube J, Wan M, Bonthuis M, Wühl E, Bacchetta J, Santos F, et al. Clinical practice recommendations for growth hormone treatment in children with chronic kidney disease. Nat Rev Nephrol 2019;15:577–89.
[Article] [PubMed] [PMC]57. Smith S, Remmington T. Recombinant growth hormone therapy for X-linked hypophosphatemia in children. Cochrane Database Syst Rev 2021;10:CD004447.
[Article] [PubMed] [PMC]58. Quinlan C, Guegan K, Offiah A, Neill RO, Hiorns MP, Ellard S, et al. Growth in PHEX-associated X-linked hypophosphatemic rickets: the importance of early treatment. Pediatr Nephrol 2012;27:581–8.
[Article] [PubMed]59. Karaplis AC, Bai X, Falet JP, Macica CM. Mineralizing enthesopathy is a common feature of renal phosphate-wasting disorders attributed to FGF23 and is exacerbated by standard therapy in hyp mice. Endocrinology 2012;153:5906–17.
[Article] [PubMed] [PMC]60. Steele A, Gonzalez R, Garbalosa JC, Steigbigel K, Grgurich T, Parisi EJ, et al. Osteoarthritis, osteophytes, and enthesophytes affect biomechanical function in adults with X-linked hypophosphatemia. J Clin Endocrinol Metab 2020;105:e1798–814.
[Article] [PubMed] [PMC]