All issues > Volume 67(12); 2024
Clinical, biochemical, and genetic study of TACE/TNF-α/ACE signaling pathway in pediatric COVID-19 infection
- Corresponding author: Mohammed H. Hassan. Department of Medical Biochemistry, Faculty of Medicine, South Valley University, 83523, Qena, Egypt Email:mohammedhosnyhassaan@yahoo.com, mohammedhosnyhassaan@med.svu.edu.eg
- Received June 26, 2024 Revised August 6, 2024 Accepted August 7, 2024
- Abstract
-
- Background
- Background
- Pediatric patients infected with coronavirus disease 2019 (COVID-19) have unique clinical characteristics. Tumor necrosis factor (TNF) is a proinflammatory cytokine that greatly contributes to tumor pathogenesis.
- Purpose
- Purpose
- To describe the presenting characteristics of COVID-19 infection among pediatric patients, and investigate the possible role of the TNF-α signaling pathway.
- Methods
- Methods
- This prospective case-control study included 50 Egyptian pediatric patients with COVID-19 and 50 healthy controls. Clinical, laboratory, and radiological assessments were performed. Serum TNF-alpha (TNF-α), TNF-α-converting enzyme (TACE), and angiotensin-converting enzyme 2 (ACE2) were measured using enzyme-linked immunosorbent assay. ACE (I/D) (rs4646994), ACE2 rs2285666, and TNF-α-308G/A single nucleotide polymorphisms (SNPs) were performed using conventional polymerase chain reaction techniques with or without restriction fragment length polymorphism.
- Results
- Results
- The median age was 1 year (interquartile range [IQR], 0.31–2.50 years) in the case group and 1.45 years (IQR, 1.00–3.00) in the control group. The main presenting symptoms were fever (92%), dry cough (74%), and dyspnea (72%). The lymphocytic count was normal in 14 patients (28%), decreased in 16 patients (32%), and increased in 20 patients (40%) of the case group. Positive chest computed tomography finding of COVID-19 infection were demonstrated among 40% of patients using COVID-19 Reporting and Data System categories (ground-glass opacity with or without consolidations in the lungs). There were significant increased serum TACE and TNF-α with decreased ACE2 levels among cases versus controls (P< 0.001). The GG genotype and G allele of the TNF-α-308G/A SNP were significantly higher in patients than in controls (P<0.05 for both), with insignificant differences in genotype and allelic frequencies in the ACE (I/D) (rs4646994) and ACE2 rs2285666 SNPs.
- Conclusion
- Conclusion
- The TNF signaling pathway was significantly activated in pediatric COVID-19 infection. Only the TNF-α-308G/A SNP was significantly associated with pediatric COVID-19 infection.
- Introduction
- Introduction
The severe acute respiratory syndrome coronavirus-2 (SARS-CoV-2) was a new beta-coronavirus that causes coronavirus disease 2019 (COVID-19) [1-3], which became a worldwide concern at the beginning of 2020 and has affected millions. COVID-19's historic appearance has not spared children. In the United States, since March 2020, about 7 million children have tested positive for the severe acute respiratory syndrome coronavirus 2 (SARS-CoV-2) [4].One of the main events in the pathophysiology of COVID-19 is the cytokine storm, which is brought on by an excess of inflammatory cytokines and causes acute respiratory distress syndrome (ARDS), a major clinical consequence of COVID-19. Cytokine release storm is triggered by tumor necrosis factor-alpha (TNF-α), one of the proinflammatory cytokines that are frequently up-regulated in acute lung damage. TNF-α-converting enzyme (TACE), a member of the family of metalloproteinase disintegrin proteins, is responsible for the conversion of inactive TNF-α precursor from to its active mature form that facilitates SARS-CoV-2 interaction with angiotensin-converting enzyme 2 (ACE2) (a receptor for SARS-CoV-2) [5].The causative agent of COVID-19, SARS-CoV-2, enters cells more easily when its spike proteins link to ACE2 receptors on host membranes. This suggests that the expression of the target ACE2 receptor in virally exposed epithelium may be associated to susceptibility to infection [6]. The related SARS-CoV-2 virus has been demonstrated to down regulate ACE2 after cell entrance, which is a factor contributing to the severe lung diseases associated with infection with this virus [7]. Compared to adults, children's serum ACE contents are higher [8]. Given that ACE and ACE2 have an inverse connection, this disparity indicates that children express ACE2 to a lesser extent than adults do. It is yet to be determined if lower levels of membrane-bound ACE2 in this population account for the low frequency of symptomatic COVID-19 in children and adolescents. The formation of TNF-α in cell culture settings has been demonstrated to be closely correlated with the SARS-CoV spike protein-induced ACE2 shedding, which may indicate the importance of regulating TNF-α in SARS-CoV [9].In the current work we explore the various clinical, laboratory, imaging characteristics, and outcome of COVID-19 among pediatric population, and for the first time to provide evidence on the possible role of TNF signaling pathway (TACE/TNF-α/ACE) in pediatric COVID-19 infection based on both biochemical and genetic assessments. Also to correlate the biochemical and genetic findings with the severity and outcome of included patients.
- Methods
- Methods
- 1. Study design and participants
- 1. Study design and participants
This was a case control observational study that was conducted at Pediatric and Chest Departments, Qena University Hospitals, in collaboration with Medical Biochemistry Departments, Faculties of Medicine, South Valley and Aswan Universities, Egypt. 50 Egyptian children who had COVID-19 and diagnosed by polymerase chain reaction (PCR) or antigen (Ag) for SARS-CoV-2 using nasopharyngeal swab, and 50 healthy (sex and age matched) children chosen as the control group between January 2021 and January 2023.Patients who were older than 18 years old, declined to participate, had a history of systemic steroid use, were on chronic medicine, or had chronic comorbidities, were excluded from the study. Each patient was presented with a recent COVID-19 diagnosis along with a severity assessment, and they were all treated medically in accordance with the guidelines for treating children with COVID-19 [10,11].The current study has been conducted in accordance to the Declaration of Helsinki. Written informed consents were obtained from parents or caregiver of the participants and the study were approved by the local Ethics Committee of Faculty of Medicine, South Valley University, Egypt. Ethical approval code (SVU.MED. PED025.2.21.5.205). To achieve 80% power and a 5% level of confidence in the results (type I error), we adjusted the sample size.- 2. Blood samples
- 2. Blood samples
Ten milliliters of venous blood was collected from each participant and each sample was divided as follows: Two milliliters of blood were evacuated into ethylenediaminetetraacetic acid (EDTA) containing tubes, and mixed thoroughly to perform complete blood count and the remaining amounts of the same sample were stored at -80°C for later genetic analysis. Two millimeters of blood were put on citrate containing tubes and then centrifuged at 3,500 rpm for 10 minutes then the measurements of D-dimer, prothrombin time (PT), prothrombin concentration (PC), and international normalized ratio (INR) were performed using the separated citrated plasma. Six milliliters of blood were allowed to clot for 30 minutes at 37°C, and then it was centrifuged for 15 minutes at 3,000 rpm and the separated sera were aliquoted into 1-mL cryotubes for measuring the following: aspartate aminotransferase (AST), alanine aminotransferase (ALT), creatinine, albumin, C-reactive protein (CRP) lactate dehydrogenase (LDH), ferritin, troponin I, SARS-CoV-2 IgG and IgM, TNF-alpha, TACE, and ACE2.- 3. Routine laboratory and imaging tools
- 3. Routine laboratory and imaging tools
1) Routine laboratory analyses include
1) Routine laboratory analyses include
(1) Complete blood picture (CBC): which was performed using Erma Automated Blood Count Machine (Tokyo, Japan); (2) Coagulation profile (PT, PC and INR), D-dimmer, and CRP were assessed using immune turbidimetry assay with the coagulation laboratory auto-analyzer (ACL 2000; Instrumentation Laboratory, Milan, Italy); (3) Serum creatinine, ALT, AST, serum albumin, and LDH were measured by using a photometric unit of the auto-analyzer the Cobas 6000 analyzer (c501 module); (4) Serum ferritin and troponin I levels were measured by TOSOH AIA-360 automated immunoassay analyzer, Japan).2) Laboratory testing for SARS-CoV-2
2) Laboratory testing for SARS-CoV-2
All patients were tested for serum SARS-CoV-2 immunoglobulins (IgG and IgM) (by immunofluorescence assay which used to semi-quantitative detection of IgM and IgG antibodies using the anti-SARS-CoV-2 IgG and IgM kits that were manufactured by Chongqing Xinsaiya Biotechnology Company in Chongqing, China), rapid SARS-COV-2 Ag nasopharyngeal testing (chromatographic immunoassay using Standard Q COVID-19 Ag test, Cat No.09cov30d; SD Biosensor Inc., Suwon, Korea), and reverse transcription quantitative PCR (RT-qPCR) confirmation from nasopharyngeal swabs (using SARS-CoV-2 Genesig Real-Time PCR Kit supplied by Primerdesign Ltd., Chandler’s Ford, UK).3) Radiological investigation
3) Radiological investigation
Nonenhanced computed tomography (CT) scan of chest was done using scanner Gantry Model CGGT-021A Japan. Severity was assessed using CO-RAD (COVID-19 Reporting and Data System).
- 4. Specific biochemical assays
- 4. Specific biochemical assays
Serum TNF-alpha, TACE, and ACE2 were measured using enzyme-linked immunosorbent assay (ELISA) assay kits that were offered commercially and provided by the Elabscience corporation, USA (catalog No.: E-EL-H0109, E-EL-H2305, and E-EL-H0281 respectively). Assays were performed using microplate ELISA reader (EMR-500, Labomed, Inc., Los Angeles, CA, USA) according to the manufacturer’s detailed instructions.- 5. PCR methods for genetic analysis
- 5. PCR methods for genetic analysis
1) ACE (I/D) (rs4646994) SNP
1) ACE (I/D) (rs4646994) SNP
It was carried out using conventional PCR and the following primer sequences without the need of a restriction enzyme: F5′CTGGAGACCACTCCCA TCCTTTCT3′;R5′GAT GTGGCCATCAC ATTCGTCAGAT-3′ according to previously published work [12,13]. The target SNP was genotyped using the following PCR conditions: the mixture was heated to 94°C for 5 minutes, followed by 35 cycles of amplification, which included denaturation at 94°C for 30 seconds, annealing at 58°C for 45 seconds, and elongation at 72°C for 30 seconds, all during the 5-minute heating period. The final extension took 5 minutes at 72°C. The D- and I alleles were identified with the presence of 190- and 490-bp fragments, respectively. The homozygous (II) genotype was considered with a 490-bp band, the homozygous (DD) genotype was interpreted with a 190-bp band, and one band at 490 bp together with a band at 190 bp demonstrate the heterozygous ID genotype (Fig. 1). Only DD genotype was presented among our study’s participants.2) ACE2 rs2285666 SNP genetic analysis
2) ACE2 rs2285666 SNP genetic analysis
The following primer sequences were used in the restriction fragment length polymorphism-PCR (RFLP-PCR) method: F5’-CATGTGGTCAAAAGGATATCT−3′)′;R5′-AAAGTA AG GTTGGCAGACAT−-3′ [14]. The following PCR conditions were applied for amplification of the target gene mutation: the mixture was heated to 95°C for 5 minutes, followed by 35 cycles of amplification, which included elongation at 72°C for 45 seconds, annealing at 51°C for 30 seconds, and denaturation at 94°C for 30 seconds. The last extension took 7 minutes at 72°C. This results in a 466-bp band size for the generated PCR products. Alu I, a restriction enzyme, was used to digest the PCR products (Catalog No. R0137S, New England Biolabs, Ipswich, MA, USA) for 4 hours at 37°C. Next, a 2% agarose gel electrophoresis was applied on the digested PCR products where the bands could be seen when exposed to ultraviolet (UV) light. Whereas allele A had 2 bands with molecular sizes of 281 bp and 185 bp, allele G was only shown one band with a molecular size of 466 bp (Fig. 2A and B).3) Genetic analysis of TNF-α-308G/A promoter polymorphism using RFLP-PCR technique
3) Genetic analysis of TNF-α-308G/A promoter polymorphism using RFLP-PCR technique
The used primers’ sequences were as follow: F5’- AGGCA ATAGGTTTTGAGGGCCAT−3′)′;R5′TCCTCCCTGCTCCGA TTCCG−3′ [15]. The following PCR conditions were employed for amplification: the mixture was heated to 95°C for 5 minutes, followed by 35 cycles of amplification, which included denaturation for 30 seconds at 94°C, annealing for 30 seconds at 60°C, and elongation for 30 seconds at 72°C. The final extension took 7 minutes at 72°C. The amplified product of the PCR was 107 bp. The restriction enzyme NcoI (Catalog No. R0193S, New England Biolabs) was used for digestion of the PCR products by incubation for 4 hours at 37°C. Then,the digested PCR products were electrophoresed in agarose gel 2% and the resulting bands were visualized under UV light. Genotype G/A was represented by 87 and 20 bp, GG homozygous genotype by 87 bp, and AA genotype by 107 bp fragments (Fig. 3).
Following the manufacturer's instructions, DNA was extracted from whole EDTA blood samples using the G-spinTM total DNA extraction kit (iNtRON Biotechnology, Inc., Seongnam, Korea). The collected DNA was stored at -80°C for the following genetic tests, which comprised:- 6. Statistical analysis
- 6. Statistical analysis
Data were analyzed using IBM SPSS Statistics ver. 26.0 (IBM Co., Armonk, NY, USA). Normality test (Kolmogorov-Smirnov & Shapiro-Wilk test) was performed. Data were presented as number, percentage, mean, standard deviation. Chi-square test and Fisher exact test were used to compare qualitative variables. Continuous data were expressed as mean±standard deviation or median and interquartile range. Differences between all groups were detected using 1-way analysis of variance test for parametric data and Kruskal-Wallis test for nonparametric data. Differences between each 2 groups were detected using independent samples T-test for parametric data and Mann-Whitney test for nonparametric data. Nominal data were expressed as percentage; differences between groups were detected using chi-square test. P value considered statistically significant when P<0.05. The studied SNPs followed Hardy-Weinberg equation. Multivariate logistic regression analysis was calculated to investigate the predictors of COVID-19 severity in children. Odds ratio (OR), 95% confidence interval (CI), and P value were calculated to evaluate the possible association of the studied SNPs with disease severity.
- Results
- Results
- 1. Demographic and clinical data of the study groups
- 1. Demographic and clinical data of the study groups
The median age of our studied cases was 1 (0.31–2.5) and it was 1.45 (1–3) of the controls group. There were 30 males (60%) and 20 females (40%) as cases while there were 22 males (44%) and 28 females (56%) controls. There were insignificant differences between cases and controls regarding age and gender distribution with P 0.05 for both. 41 patients (82%) have mild to moderate disease, while the remaining 9 patients (18%) have severe to critical COVID-19.The most common presenting symptoms in children with COVID-19 were fever (92%), dry cough (74%), and dyspnea (72%). Vomiting was experienced by 11 patients (22%).Eight patients (16%) had diarrhea. Just 2 patients (4%), reported having abdominal pain. There were renal manifestations in 2 patients (4%), neurological manifestations in 9 patients (18%), convulsions in 6 patients (12%) and disturbed conscious level in 4 patients (8%) as described in (Table 1).- 2. Routine laboratory and radiological data of the studied groups
- 2. Routine laboratory and radiological data of the studied groups
Twenty-two patients (44%) tested positive for SARS-CoV-2 IgG, 34 (68%) tested positive for SARS-CoV-2 IgM, 10 (20%) tested positive for SARS-CoV-2 Ag, and 5 (10%) tested positive for SARS-CoV-2 by PCR.There were statistically significant (P<0.001) decreased hemoglobin, mean corpuscular volume and mean corpuscular hemoglobin values in cases group when compared with control group. There was anemia in 31 patients (62%) of cases group. Anemia was Macrocytic in 1 patient (2%), microcytic in 29 patients (58%), and microcytic normochromic in 1 patient (2%), normocytic in 1 patient (2%) of cases group. Platelets counts were normal in 25 patients (50%), decreased in 3 patients (6%) and increased in 22 patients (44%) of cases group. White blood cells (WBCs) counts were normal in 16 patients (32%), decreased in 3 patients (6%) and increased in 31 patients (62%) of cases group. Lymph/total WBCs % was normal in 14 patients (28%), decreased in 16 patients (32%) and increased in 20 patients (40%) of cases group. Neutrophil/total WBCs % was normal in 18 patients (36%), decreased in 11 patients (22%) and increased in 21 patients (42%) of cases group, as described in (Table 2).Among cases, coagulation profile (PT, PC and INR) was impaired in 9 patients (18%). There were 26 patients (52%) with positive CRP. Ferritin was normal in 30 patients (60%), decreased in 1 patient (2%) and increased in 19 patients (38%). LDH was normal in 38 patients (76%), decreased in 1 patient (2%), and increased in 11 patients (22%). Troponin I was normal in 24 patients (48%) and increased in 26 patients (52%). D-Dimer was normal in 29 patients (58%), decreased in 18 patients (36%) and increased in 3 patients (6%). There were a highly statistically significant increased PT, ferritin and LDH (P<0.001) in cases group when compared with control group as presented in (Table 2).Serum creatinine was normal in 44 patients (88%), low in 5 patients (10%) and elevated in 1 patient (2%). Serum albumin was normal in 42 patients (84%) and increased in 8 patients (16%) and it showed no statistically significant difference (P=0.136) between studied groups (cases and control). AST was normal in 31 patients (62%) and increased in 19 patients (38%) of cases group. ALT was normal in 42 patients (84%) and increased in 8 patients (16%). There were statistically significant increased AST and ALT levels (P<0.001) in cases (median were 33 [24.75–62] and 28 [19–40.25], respectively) when compared with control group (median durations were 20 [16–25], and 18 [12–20], respectively) as illustrated in (Table 2).There were positive CT chest findings for COVID-19 in 20 pediatric patients infected with COVID-19 (40%). There were 2 patients (10%) with CO-RAD 3, 7 patients (35%) with COVID-19 Reporting and Data System grade 4, 10 patients (50%) with COVID-19 Reporting and Data System grade 5 and 1 patient (5%) with COVID-19 Reporting and Data System grade 6.- 3. Circulating biochemical markers of TNF signaling pathway among the participants
- 3. Circulating biochemical markers of TNF signaling pathway among the participants
There were a statistically significant (P<0.001) increased median levels of both TNF converting enzyme and TNF-alpha with statistically significant lower median ACE2 levels among cases (4,377.19 [2,337.09–5,558.90] pg/mL, 31.29 [27.42–35.62] pg/mL, and 12.98 [5.83–26.32] ng/mL, respectively) compared to the control group (462.96 [113.01–2,892.23], 1.87 [0.77–3.05], and 37.43 [34.09–40.74], respectively). Insignificant differences regarding to serum TNF converting enzyme, TNF-alpha, and ACE2 levels among pediatric patients with COVID-19 in term of disease severity as described in (Table 3). There were insignificant correlations between the serum levels of TNF converting enzyme, TNF-alpha, and ACE2 with each other among the included patients, P>0.05 for all.- 4. Genotypes and alleles frequencies of the studied SNPs related to TNF signaling pathway among the study groups
- 4. Genotypes and alleles frequencies of the studied SNPs related to TNF signaling pathway among the study groups
Regarding to ACE (I/D) (rs4646994) SNP, only DD genotype was presented among all participants, while the ID and II genotypes did not exist, meaning that this SNP was not being investigated for further statistical analysis.Regarding to ACE2 (rs2285666) SNP, there were no statistically significant difference in the genotypes or alleles frequencies between cases and controls, and between the patients’ subgroups in term of COVID-19 severity (P>0.05 for all), as presented in (Table 4).As regards TNF-α-308G/A promoter SNP, GG genotype frequency was considerably higher in cases (66%) than in controls (40%), with a P value of 0.026. According to the dominant model, the frequency of (GA+AA) genotypes was significantly lower in cases (34%) than in the control group (60%), with a P value of 0.009. Compared to the controls (67 %), pediatric COVID-19 patients had a substantially greater frequency of the G allele (80%), P=0.037. with OR=1.970 at 95% CI (1.035–3.749). This indicates that G allele can be considered as a genetic risk factor associated with SARS-CoV-2 infection among pediatric patients as illustrated in (Table 5). In terms of COVID-19 severity among the included pediatric patients, there were insignificant differences in the frequencies of both genotypes and alleles of TNF-α-308G/A promoter SNP between pediatric patients with mild to moderate COVID-19 and those with severe to critical disease, P>0.05 for all, (Table 5).Additionally, there were insignificant differences regarding median serum TNF converting enzyme, TNF-α, and ACE2 levels in terms of genotypes and alleles of ACE2 rs228 5666 and TNF-α-308G/A promoter SNPs, P>0.05 for all.- 5. Predictors of COVID-19 severity among pediatric patients
- 5. Predictors of COVID-19 severity among pediatric patients
In order to investigate the predictors of severity in children with COVID-19, logistic regression analysis was employed and revealed that dyspnea (P=0.012) and its duration (P=0.037), fever (P=0.005), total disease duration (P=0.020), and platelet count (P=0.025) were significant predictors of COVID-19 infection severity among pediatric patients.- 6. Outcome of COVID-19 among the included pediatric patients
- 6. Outcome of COVID-19 among the included pediatric patients
There were 48 patients (96%) with complete recovery and 2 patients (4%) developed complications and were placed on mechanical ventilation (1 patient [2%] developed respiratory failure and the other [2%] developed respiratory failure and die).
- Discussion
- Discussion
The intricate relationship between the host, virus, and environment that dictates the severity of COVID-19 infection in children leads to varying clinical courses and disease outcomes. To pinpoint the precise features of pediatric SARS-CoV-2 infection, there is insufficient evidence. This research provided COVID-19 demographic, clinical, laboratory, radiological, and genetic features among a cohort of pediatric patients from Egypt.The majority of COVID-19 infected cases in our study (IQR, 0.31–2.50 years) were seen in infants, which is consistent with other publications [16]. This can be the case because they need certain safety precautions and are unable to wear masks. Additionally, we discovered a predominance of male gender, which is in line with earlier research on adults and children that revealed male youngsters had a tendency to be more vulnerable to COVID-19 [17-19]. While there was no statistically significant difference between both sexes in this research, it is possible that males' higher expression levels of ACE2 or genetic and immunological variations contribute to females' decreased susceptibility to the infection [18]. In contrast to our results Sahin et al. [20] found that the median age was 72 months (2–216 months), among which 102 (53.7%) were females. Another study by Ali [21]. found that the median age of the studied patients was 6 (IQR, 2–10) years and a previous meta-analysis by Amenomori et al. [22], including 203 children, also found that the mean age of the studied patients was 5.46 years.Regarding symptoms at presentation, we agree with previous researches in which fever and cough were the most prevalent clinical characteristics of COVID-19 among children (present in 92% and 74%, respectively, of the total of our symptomatic children) [23-26]. In contrast to our study, Wang et al. [27], found that fever and cough occurred only in 48% and 39% of children, respectively which indicates that fever and cough in children are not as common as in adults. In our study fever present in 92% of patients and its duration ranges from 2 to 10 days with its median 5 days which agree to findings of a study by Wang et al. [27], who reported 32.3% of patients complained of fever over 38°C, and 32.3% had a mild fever of 37.3°C–38°C. The fever lasted 1–9 days and subsided within 3 days in 75% of patients. Jiehao et al. [28] found that, all patients had fever over 39°C (duration, 3–11 days; median, 6 days) and cough. In addition to respiratory symptoms, a significant number of patients experienced neurological or gastrointestinal symptoms as the first and predominant COVID-19 symptoms in children in this study (22% and 18%, respectively), while a small percentage of patients experienced renal manifestations (4%). We observed that the rate of gastrointestinal complaints was significantly higher than in adult researches [29,30]. Also digestive symptoms are not infrequent as some publications have already exhibited [31,32]. Children with COVID-19 infection who initially experience gastrointestinal symptoms need special attention. Despite the fact that children with COVID-19 infection are less likely to experience gastrointestinal symptoms, previous researchers have discovered SARS-CoV-2 particles in the patients'faeces [33,34], and according to a previously published study, there may be a chance of fecal-oral transmission because certain children continued to test positive on rectal swabs even after nasopharyngeal testing came back negative [35]. In 1 research of 31 children, diarrhea was the initial symptom in 3 of them [31]. Furthermore, without any evident respiratory tract infection in the early stages, 3 of the 8 children with severe COVID-19 infection had gastrointestinal symptoms that were obviously present, and one of them had gastrointestinal symptoms from the beginning without respiratory manifestations [36]. Further research is necessary to determine the function of fecal-oral transmission, as evidenced by the prolonged shedding of viral material in fecal samples identified by PCR testing in numerous instances. The percentage of hospitalized children in our group who were on mechanical ventilation (4%) was comparable to 2 earlier studies (7% and 6%) [37,38], although less than in Belay et al. [39] (14.6% in the COVID-19 cohort).In the current study, positive immunoglobulin tests (IgG and IgM) for SARS-CoV-2 were found in 44% and 68% respectively, while positive SARS-CoV-2 Ag was found in 20%, and finally nasopharyngeal SARS-CoV-2 molecular testing (RT-PCR) was positive in only 10% of clinically symptomatic children. In a previous study by Nyabi et al. [40], positive IgM, IgG and both IgM and IgG were detected in 4%, 2% and 1.2% respectively. In a study by Jia et al. [41], the SARS-CoV-2 nucleic acid positivity rate was 42.1 %, but the IgM and IgG results were positive in all patients. For 1 year, the SARS-CoV-2 antibody detection methods including an enzyme-linked immunosorbent assay, a rapid immunochromatographic assay, and a chemiluminescent immunoassay has been applied and its clinical value is being evaluated by Lee et al. [42], which concluded that compared with nucleic acid detection, IgM and IgG detection may provide a quick, simple, and accurate detection method for suspected COVID-19 cases. IgM and IgG antibody detection can identify suspected cases with negative nucleic acid tests. Diagnostic accuracy of COVID-19 might be improved by nucleic acid testing in patients with a history of epidemic disease or with clinical symptoms, as well as CT scans when necessary, and serum-specific IgM and IgG antibody testing after the window period. Ag test positivity was lower before and on the day of illness onset. This is notable, as SARS-CoV-2 transmission often occurs before and during the first few days following symptom onset [43-45]. This may give explanation why our Ag results were relatively low among the studied patients.With respect to regular laboratory results of COVID-19 infection in pediatric patients, a prior meta-analysis conducted by Wang et al. [46] discovered that children with COVID-19 typically had leukocyte counts that were normal or below normal ranges and lymphocyte counts that were normal or above normal ranges. According to our analysis, the leukocyte count was often either normal (32%) or above the normal range (62%) and the lymphocyte count was either low (32%) or raised (40%). Still, a decrease in lymphocyte count is one of the variables used in some published COVID-19 guidelines and agreement for pediatric patients to diagnose suspected cases [47,48]. Our research indicated that recommendations for children shouldn't be created strictly according to adult norms, as this could lead to the omission of real cases. Many explanations for the lymphopenia observed in COVID-19 infected patients have been put forth, such as the virus's direct impact on lymphocyte recruitment, the lymphatic organs' damage, and the existence of an inflammatory cytokine storm [49]. The findings displayed here were consistent with earlier research [16,50,51], and contradictory with adults, since lymphopenia in children with COVID-19 infection cannot be regarded as a helpful sign. Our research defied expectations by showing that lymphocytosis was more common in children than in adults and was more noticeable in pediatric patients than lymphopenia.In our study elevated levels of CRP, serum ferritin, LDH and troponin I were reported in considerable percentages of the studied patients as follow 52%, 38%, 22% and 52% respectively. This is not new and consistent with a recent study by Zhu et al. [52], who showed elevated levels of CRP, serum ferritin, and LDH in patients with COVID-19 infection. It has been established that CRP plays a part in controlling infection and is used for evaluating inflammation in the body [53]. The results of our research showed that 52% of patients exhibited positive CRP.D-dimer was only increased in 6% of our studied patients. Our results were in contrast to findings in adult studies which reported that the hypercoagulable state linked to COVID-19 is the cause of the increases in D-dimer levels [54,55]. Also multiple studies have identified an association between higher D-dimer levels and an increased risk of mortality in the COVID-19 infected patient population [56,57]. This may be explained by the infrequent severe presentation among the children compared to adult.Severe liver dysfunction is unusual; most liver injuries are mild and resolve with time. The current study reported elevated ALT and AST in 16% and 38% respectively while serum albumin was decreased in 16% of the studied patients. Previous studies reported liver damage in children with COVID-19 infection as shown by 13% to 50% of individuals having increased ALT and AST blood values [58-60]. The severity of COVID-19 has also been associated with abnormal transaminase levels; liver injury was observed in 58.8% of individuals with moderate disease and 66.7% of those with severe illness [61,62].Only 2% of the participants in the study experienced high creatinine levels, indicating that our patients had a very low risk of acute kidney injury (AKI). There were very few pediatric researches explained the risk of AKI in children infected with COVID-19. Cheng et al. [63] reported elevation of serum BUN and creatinine in 15.5% and 14.17% of patients, respectively. High and persistent fever, inadequate hydration, and a delay in care center referrals are possible causes. A study by Sorkhi et al. [64] evaluated the kidney manifestation in children infected with COVID-19 showed that the risk of AKI was considerably high in the studied patients. It reported that 66% of children had GFR less than 90 mL/min/1.73 m2 and one quarter of children had GFR less than 60 mL/min/1.73 m2. Of them, 2 cases needed replacement therapy (hemodialysis) for about one week. There were not any prior kidney diseases in these children.Given that symptoms in children infected with COVID-19 are reasonable, a chest CT scan appears to be a more dependable procedure than a chest x-ray. Forty percent of pediatric patients in our study exhibited ground-glass opacities in their CT chest images. According to a research by Oterino Serrano et al. [65], 90% of pediatric patients showed ground-glass opacities. Notwithstanding the limitations resulting from the limited number of CT findings, our results were consistent with earlier research showing that the chest CT can be an effective way to investigate pneumonia in children with COVID-19 infection, even before clinical symptoms manifest [66].The overproduction of inflammatory cytokines, known as cytokine storm, is a crucial step in the pathogenesis of COVID-19. It is the primary cause of ARDS, a common clinical consequence linked to the virus. Our study showed that among the studied pediatric patients, TNF-α converting enzyme and TNF-α serum levels were significantly higher than controls, while serum ACE2 level was significantly lower. Our results, which showed that COVID-19 infected patients had greater TNF-α levels, were supported by some earlier research [67.68]. In one study, Alabd and Mahmoud [69] found that serum TNF-α levels are not a significant biomarker for the diagnosis or prognosis of patients with mild COVID-19 infection. In contrast, Han et al. [70] found that the levels of TNF-α, IL-6, IL-2, IL-10, and IFN-γ were higher in COVID-19 patients than in healthy subjects.A study by Bani Hani et al. [71] showed that the critically ill and nonsurvivor patients had significantly higher ACE2 levels, but there was no difference in ACE2 levels between the COVID-19 positive group and the control group. This suggests that elevated ACE2 levels may be a predictor of disease severity. The majority of the children infected with COVID-19 who were included in our study had mild to moderate illness, which could account for the significantly lower levels of ACE2 in our patients.After genotype testing of TNF-α-308G/A promoter SNP, the G allele was significantly more expressed in patients vs. controls. 66% of patients were GG, 14% were GA, and 6% were AA versus 40%, 54% and 6% in the control group respectively. With marginal P value (P=0.051), GG genotype was associated with more aggressive disease in comparison to the other genotypes. In the GG genotype, the disease was severe in (66.6%) cases in comparison to (22.2%) cases in the AA genotype and (11.1%) cases in the GA genotype. This indicates that persons carrying the G allele are more susceptible to SARS-CoV-2 infection. This result was not consistent with a study by Saleh et al. [72] who found A allele is more expressed in patients vs. controls with and that individuals with AA genotypes are more susceptible to COVID- 19. Our findings were also similar to a study by Sotomayor-Lugo et al. [73] who found that AA genotype of TNF-α-308G/A promoter SNP may not be a relevant factor in severe forms and the A allele was low in individuals with severe forms of the disease and with the diseased individuals in general.There are many genetic variations within both the ACE and ACE2 receptor genes, such as the functional polymorphism of I/D in the ACE receptor gene and the rs2106809 and rs2285666 polymorphisms in the ACE2 receptor gene. The ACE2 receptor gene polymorphisms impact the amount of circulating ACE2 receptors, and the D allele exhibits increased ACE activity [74,75]. Considering the role of ACE in COVID-19 pathogenesis and the variation in disease severity, ACE I/D, and ACE2 receptor gene variants have attracted the attention of researchers.To the best of our knowledge, by the genotype testing of ACE I/D, only DD genotype could be detected in our study among participants while ID and II genotypes were absent indicating lack of significant role of ACE SNP in pediatric COVID-19 infection. Future research is necessary to validate the results of our research because no prior studies regarding the potential role of ACE I/D SNP in pediatric COVID-19 infection could be found in the literature. Additionally, in the current work, genotype testing of ACE2 (rs2285666) SNP revealed insignificant differences between the patients and controls as regards the genotypes and alleles distribution, but the G allele was more frequent in patients, and 68% of patients were GG genotype. This indicates that children carrying the G allele are more vulnerable to SARS-CoV-2 infection. Also, GG genotype was more frequent in severe to critical COVID-19 pattern in comparison to the other genotypes. According to Möhlendick et al. [76] who also discovered that the GG genotype or G allele of ACE2 (rs2285666) SNP was substantially related with a nearly two-fold greater risk of contracting SARS-CoV-2 infection and a three-fold increased risk of developing serious disease, their findings are consistent with our own.A proper understanding of the leading causes of severe illness may decrease the disease burden and fatality rates through early management to prevent serious complications. To the best of our knowledge, this is one of the few studies that looked at the characteristics of children and adolescents with severe form of SARS-CoV-2 infection in our community. We analyzed demographic, clinical, immunological, hematological, biochemical, genetic, and radiographic findings that may be of utility to clinicians in predicting COVID-19 severity and mortality. Dyspnea and fever were significant predictors for COVID-19 severity in our study which was in agreement with Ng et al. [77]. In line with our findings, a meta-analysis research [78], who found that dyspnea and tachypnea significantly associated with the risk of severe illness. Also, dyspnea was a significant risk factor for severe COVID-19 infection among children as demonstrated by Graff et al. [79] It was surprising to find that, in line with a previous study by Najafinejad et al. [17], common laboratory markers like CRP and lymphopenia do not seem to be significant predictors of the severity of illness among children infected with COVID-19. Nevertheless, more research is required to validate the results of this study. In contrast to our findings, a recent study [80] reported that increasing white blood cell count, younger age, hypoxia, and bilateral infiltrates on chest radiographs at admission were independent predictors of severe disease. Another pediatric registry [81] conducted in Asia revealed that the risk factors for severe disease in children were age younger than 12 months, presence of comorbidities, and cough at presentation.No significant correlation was found in our investigation between the severity of the disease and the CT findings. Similarly, a study by Guo et al. [82] demonstrated that the symptoms and severity of pediatric COVID-19 patients were not predicted by an abnormal chest imaging. On the other hand, a recent research of 76 pediatric patients with proved COVID-19 infection revealed a significant association between the COVID-19 severity and the chest CT score, indicating that it can be used to measure the severity of the illness [83]. Furthermore, we found no proof that the severity of COVID-19 was significantly correlated with variations in the serum levels of TNF-α converting enzyme, TNF-α, ACE2, or genetic variations in TNF-α, or ACE2 genes. The discrepancies in age groups, clinical presentations, and severity evaluation techniques across the populations under study could account for the differences in severity predictions between our study and the prior research.Ninety-six percent of the children in our study who had COVID-19 infection had fully recovered, indicating that the prognosis for these individuals was generally favorable. In our study, 82% of the pediatric COVID-19 infected patients had mild to moderate disease. Eighteen percent of our patients were experiencing severe and serious illness. During the course of our study, one patient (2% of the pediatric patients with COVID-19-associated fatalities) died. This was in line with earlier research that showed no or very infrequent child fatalities [84-86]. Also, earlier publications highlighted the low child death rates [81,87], and during the course of the pandemic's 2 years, Malaysia had a low number of pediatric mortality [88]. Respiratory failure was the study's documented primary cause. Also, Ruan et al. [89] claimed that the primary cause of mortality for COVID-19 infected individuals is respiratory failure related to ARDS. The findings of our study are in accordance with other published studies Castagnoli et al. [90] and Ludvigsson [16], who reported that children have a better prognosis for COVID-19, when compared to adults, which was explained by the difference in the immune system response to viral infection [16,91].Our study was limited in a number of ways. It was a single-center study at first. Second, this study's small sample size reduced generalizability. Moreover, children might not be able to articulate their symptoms precisely, and patients may not disclose their problems. Therefore, to validate our findings, more multicentric longitudinal studies with a greater number of patients should be conducted.In conclusion, children with SARS-CoV-2 frequently had fever, cough, dyspnea, lymphocytosis, normal ferritin, LDH, and D-dimmer levels. Pediatric patients infected with COVID-19 showed significant activation of the TNF signaling pathway. The only SNP that showed a significant correlation with pediatric COVID-19 was TNF-α-308G/A. The majority of pediatric COVID-19 infected patients recovered fully, and their prognosis was favorable.
- Footnotes
-
Conflicts of interest No potential conflict of interest relevant to this article was reported.
Funding This work was partially funded by Faculty of Medicine, South Valley University, 83523, Qena, Egypt.
Author contribution Conceptualization: MHH, AEAA, NIR, EAAE, HSS , HM; Data curation: MHH, AEAA, NIR, EAAE, HSS, HM, SGR, SMAA; Formal analysis: MHH, AEAA, NIR, EAAE, HSS, HM, SGR, SMAA; Funding acquisition: MHH, AEAA, NIR, EAAE, HSS, HM, SGR, SMAA; Methodology: MHH, AEAA, NIR, EAAE, HSS, HM, SGR, SMAA; Project administration: MHH, AEAA, NIR, EAAE, HSS , HM, SGR, SMAA; Visualization: MHH, AEAA, NIR, EAAE, HSS, HM, SGR, SMAA; Writing-original draft: MHH; Writing-review & editing: MHH, AEAA, NIR, EAAE, HSS , HM, SGR, SMAA
-
Fig. 1.
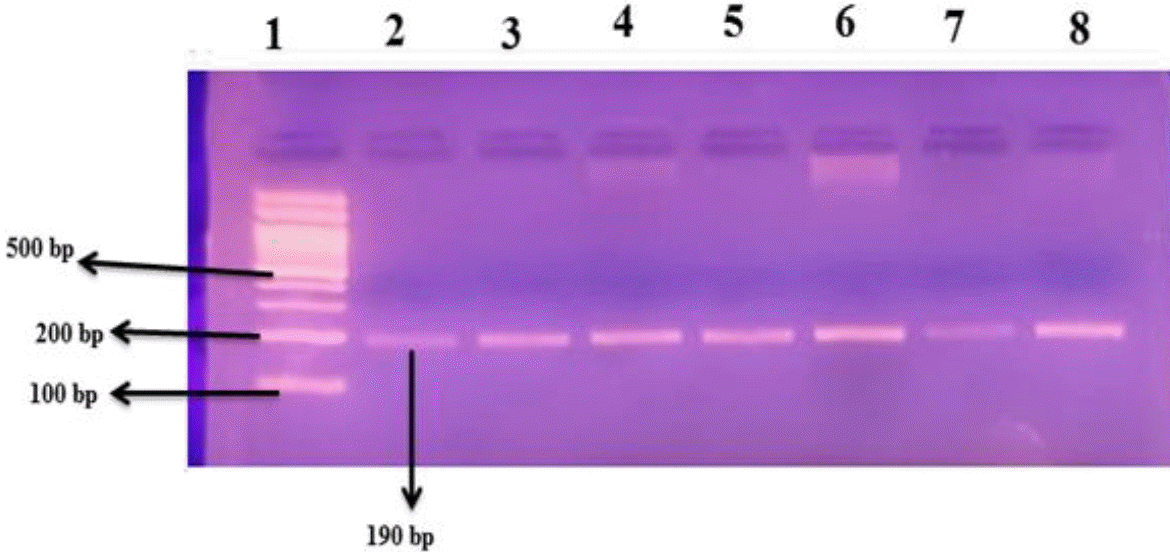
Fig. 2.
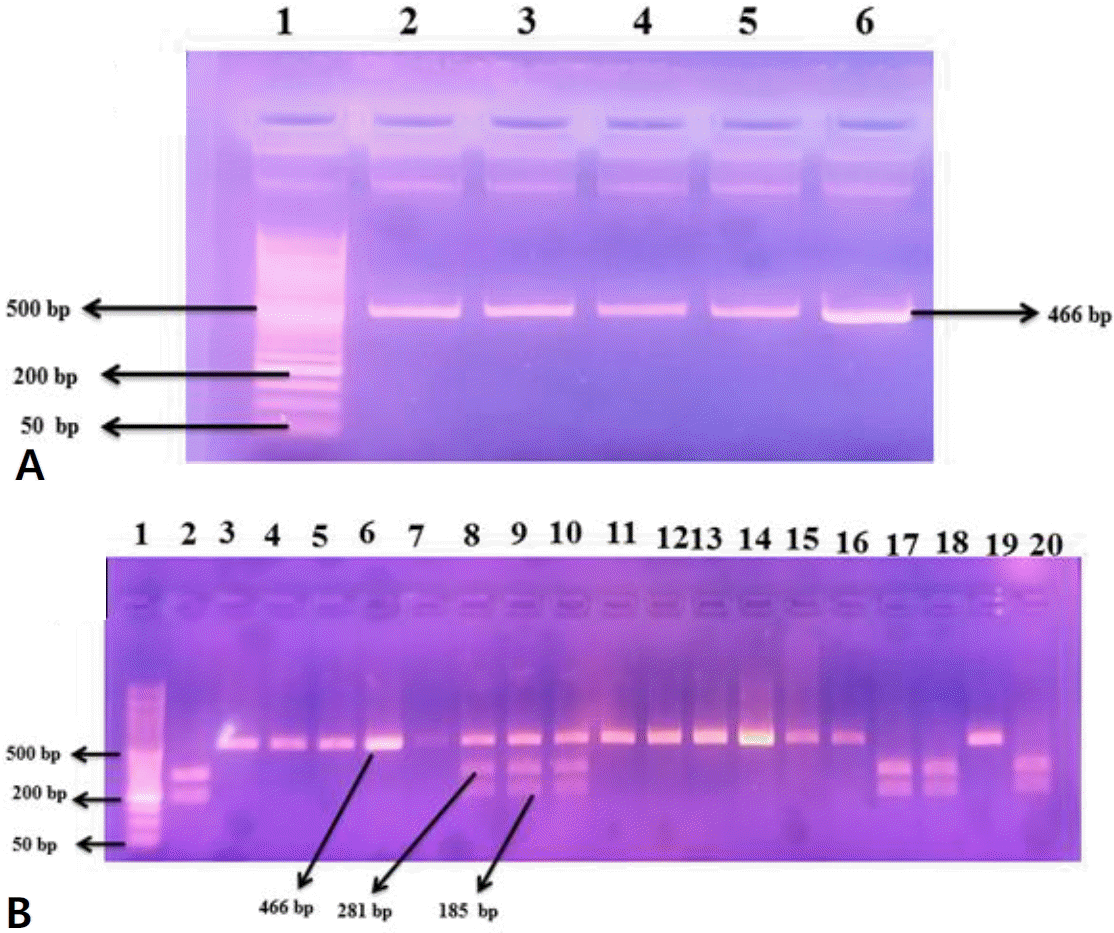
Fig. 3.
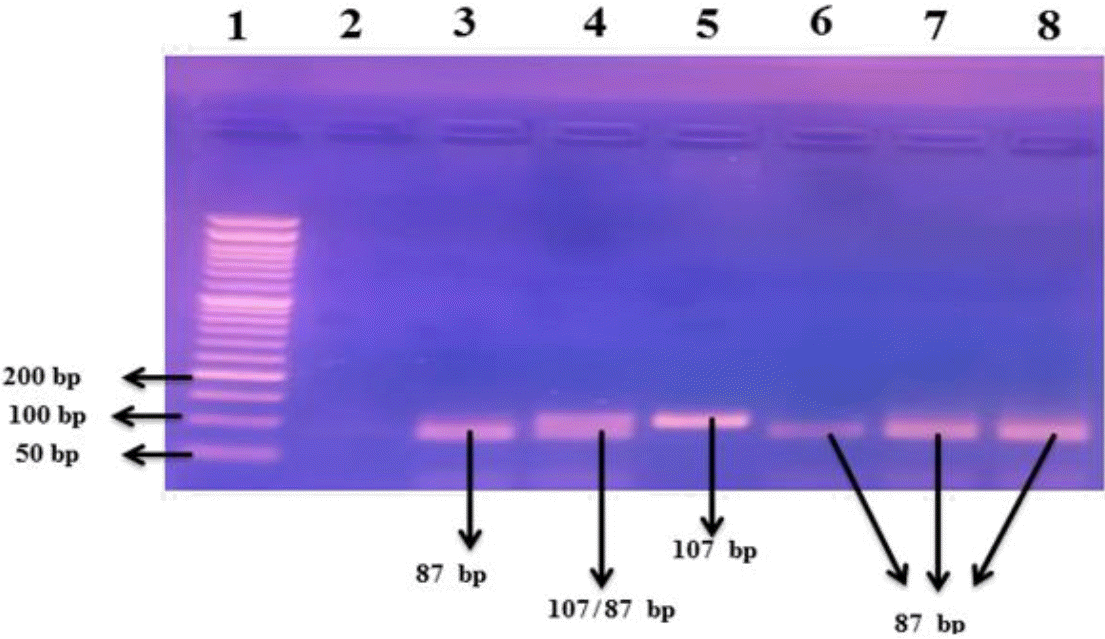
Table 1.
Table 2.
Values are presented as mean±standard deviation or median (interquartile range).
Hb, hemoglobin; RBC, red blood cell count; MCV, mean corpuscular volume; MCH, mean corpuscular hemoglobin; RDW, red cell distribution width; HCT, hematocrit; PLT, platelets; WBC, white blood cell; PT, prothrombin time; PC, prothrombin concentration; INR, international normalized ratio; CRP, C-reactive protein; LDH, lactate dehydrogenase; AST, aspartate aminotransferase; ALT, alanine aminotransferase.
Boldface indicates a statistically significant difference with P <0.05.
Table 3.
Table 4.
Table 5.
Group |
TNF-α-308G/A promoter SNP genotypes |
TNF-α-308G/A promoter SNP alleles |
|||||||
---|---|---|---|---|---|---|---|---|---|
GG | GA | AA | GG+GA | AA | GG | GA+AA | G | A | |
Study groups | |||||||||
Cases (n=50) | 33 (66) | 14 (28) | 3 (6) | 47 (94) | 3 (6) | 33 (66) | 17 (34) | 80 (80) | 20 (20) |
Controls (n=50) | 20 (40) | 27(54) | 3 (6) | 47 (94) | 3 (6) | 20 (40) | 30 (60) | 67 (67) | 33 (33) |
P value (χ2) | 0.026* (7.311) | 1.000 (0.000) | 0.009* (6.784) | 0.037* (4.338) | |||||
OR (95% CI) | - | 1.000 (0.192–5.210) | 2.912(1.290–6.571) | 1.970 (1.035–3.749) | |||||
Patients’ subgroups | |||||||||
Mild to moderate (n=41) | 27 (65.9) | 13 (31.7) | 1 (2.4) | 40 (97.6) | 1 (2.4) | 27 (65.9) | 14 (34.1) | 67 (81.7) | 15 (18.5) |
Severe to critical (n=9) | 6 (66.7) | 1 (11.0) | 2 (22.2) | 7 (77.8) | 2 (22.2) | 6 (66.7) | 3 (33.3) | 13 (72.2) | 5 (27.8) |
P value (χ2) | 0.051 (5.933) | 0.080 (5.121) | 0.963 (0.002) | 0.362 (0.830) | |||||
OR (95% CI) | - | 11.429 (0.909–143.642) | 0.964 (0.209–4.448) | 1.718 (0.531–5.555) |
- References
- 1. Ludwig S, Zarbock A. Coronaviruses and SARS-CoV-2: a brief overview. Anesth Analg 2020;131:93–6.
[Article] [PubMed] [PMC]2. Hu B, Guo H, Zhou P, Shi ZL. Characteristics of SARS-CoV-2 and COVID-19. Nat Rev Microbiol 2021;19:141–54.
[Article] [PubMed] [PMC]3. Mohandas S, Yadav PD, Shete A, Nyayanit D, Sapkal G, Lole K, et al. SARS-CoV-2 delta variant pathogenesis and host response in Syrian hamsters. Viruses 2021;13:1773.
[Article] [PubMed] [PMC]4. Falk A, Benda A, Falk P, Steffen S, Wallace Z, Høeg TB. COVID-19 Cases and transmission in 17 K–12 Schools — Wood County, Wisconsin, August 31–November 29, 2020. MMWR Morb Mortal Wkly Rep 2021;70:136–40.
[Article] [PubMed] [PMC]5. Lambert DW, Yarski M, Warner FJ, Thornhill P, Parkin ET, Smith AI, et al. Tumor necrosis factor-alpha convertase (ADAM17) mediates regulated ectodomain shedding of the severe-acute respiratory syndrome-coronavirus (SARS-CoV) receptor, angiotensin-converting enzyme-2 (ACE2). J Biol Chem 2005;280:30113–9.
[PubMed]6. Wan Y, Shang J, Graham R, Baric RS, Li F. Receptor recognition by the novel coronavirus from Wuhan: an analysis based on decade-long structural studies of SARS coronavirus. J Virol 2020;94:e00127–20.
[Article]7. Kuba K, Imai Y, Rao S, Gao H, Guo F, Guan B, et al. A crucial role of angiotensin converting enzyme 2 (ACE2) in SARS coronavirus-induced lung injury. Nat Med 2005;11:875–9.
[PubMed] [PMC]8. Rodriguez GE, Shin BC, Abernathy RS, Kendig EL Jr. Serum angiotensin-converting enzyme activity in normal children and in those with sarcoidosis. J Pediatr 1981;99:68–72.
[Article] [PubMed]9. Haga S, Yamamoto N, Nakai-Murakami C, Osawa Y, Tokunaga K, Sata T, et al. Modulation of TNF-alpha-converting enzyme by the spike protein of SARS-CoV and ACE2 induces TNF-alpha production and facilitates viral entry. Proc Natl Acad Sci U S A 2008;105:7809–14.
[PubMed] [PMC]10. Mostafa AS, Abdalbaky A, Fouda EM, Shaaban HH, Elnady HG, Hassab-Allah M, et al. Practical approach to COVID-19: an Egyptian pediatric consensus. Egypt Pediatric Association Gaz 2020;68:28.
[Article]11. Rizk SG, Ahmed AE, Hassan MH, Rashwan NI. Characteristics and outcomes of COVID-19 in pediatric patients with chronic diseases attending Qena University Hospital, Upper Egypt. SVU Int Med Sci 2024;7:62–81.
[Article]12. Zmorzynski S, Szudy-Szczyrek A, Popek-Marciniec S, Korszen-Pilecka I, Wojcierowska-Litwin M, Luterek M, et al. ACE insertion/deletion polymorphism (rs4646994) is associated with the increased risk of multiple myeloma. Front Oncol 2019;9:44.
[Article] [PubMed] [PMC]13. Bekiet MA, Elbeh KA, Abbas MA, Hassan MH, Sakr SF, Desoky T. Genetic profile of ACE (I/D) (rs4646994) single nucleotide polymorphism among sample of Egyptian patients with Alzheimer disease in upper Egypt. SVU Int Med Sci 2023;6:684–94.
[Article]14. Mahmood ZS, Fadhil HY, Abdul Hussein TA, Ad'hiah AH. Severity of coronavirus disease 19: Profile of inflammatory markers and ACE (rs4646994) and ACE2 (rs2285666) gene polymorphisms in Iraqi patients. Meta Gene 2022;31:101014.
[Article] [PubMed] [PMC]15. Kaur A, Kaur A. Recurrent pregnancy loss: TNF-α and IL-10 polymorphisms. J Hum Reprod Sci 2011;4:91–4.
[Article] [PubMed] [PMC]16. Ludvigsson JF. Systematic review of COVID-19 in children shows milder cases and a better prognosis than adults. Acta Paediatr 2020;109:1088–95.
[Article] [PubMed] [PMC]17. Najafinejad M, Cheraghali F, Aghcheli B, Rajabi A, Barati L, Naziri H, et al. COVID-19 in pediatrics: demographic, clinical, laboratory, and radiological characteristics of infected patients with SARS-CoV-2. Front Pediatr 2022;9:808187.
[Article] [PubMed] [PMC]18. Samadizadeh S, Masoudi M, Rastegar M, Salimi V, Shahbaz MB, Tahamtan A. COVID-19: why does disease severity vary among individuals? Respir Med 2021;180:106356.
[Article] [PubMed] [PMC]19. Takahashi T, Ellingson MK, Wong P, Israelow B, Lucas C, Klein J, et al. Sex differences in immune responses that underlie COVID-19 disease outcomes. Nature 2020;588:315–20.
[PubMed] [PMC]20. Sahin A, Dalgic N, Sancar M, Congur EC, Kanik MK, Ilgar SD, et al. Pediatric patients with COVID-19: a retrospective single-center experience. Sisli Etfal Hastan Tip Bul 2022;56:62–9.
[Article] [PubMed] [PMC]21. Ali HA. Major determinant factors of pediatric COVID-19 severity; a single center study. Egypt Pediatric Association Gaz 2023;71:22.
[Article] [PMC]22. Amenomori M, Mukae H, Sakamoto N, Kakugawa T, Hayashi T, Hara A, et al. HSP47 in lung fibroblasts is a predictor of survival in fibrotic nonspecific interstitial pneumonia. Respir Med 2010;104:895–901.
[Article] [PubMed]23. Patel NA. Pediatric COVID-19: systematic review of the literature. Am J Otolaryngol 2020;41:102573.
[Article] [PubMed] [PMC]24. Hoang A, Chorath K, Moreira A, Evans M, Burmeister-Morton F, Burmeister F, et al. COVID-19 in 7780 pediatric patients: a systematic review. EClinicalMedicine 2020;24:100433.
[Article] [PubMed] [PMC]25. Del Hoyo Soriano L, Sterling A, Edgin J, Hamilton DR, Berry-Kravis E, Dimachkie Nunnally A, et al. Associations among sex, cognitive ability, and autism symptoms in Individuals with Down syndrome. J Autism Dev Disord 2024;54:301–11.
[Article] [PubMed] [PMC]26. King JA, Whitten TA, Bakal JA, McAlister FA. Symptoms associated with a positive result for a swab for SARS-CoV-2 infection among children in Alberta. CMAJ 2021;193:E1–9.
[Article] [PubMed] [PMC]27. Wang D, Ju XL, Xie F, Lu Y, Li FY, Huang HH, et al. Clinical analysis of 31 cases of 2019 novel coronavirus infection in children from six provinces (autonomous region) of northern China. Zhonghua Er Ke Za Zhi 2020;58:269–74.
[PubMed]28. Jiehao C, Jin X, Daojiong L, Zhi Y, Lei X, Zhenghai Q, et al. A case series of children with 2019 novel coronavirus infection: clinical and epidemiological features. Clin Infect Dis 2020;71:1547–51.
[Article] [PubMed] [PMC]29. Chen F, Liu ZS, Zhang FR, Xiong RH, Chen Y, Cheng XF, et al. First case of severe childhood novel coronavirus pneumonia in China. Zhonghua Er Ke Za Zhi 2020;58:179–82.
[PubMed]30. Huang W, Li C, Wang Z, Wang H, Zhou N, Jiang J, et al. Decreased serum albumin level indicates poor prognosis of COVID-19 patients: hepatic injury analysis from 2,623 hospitalized cases. Sci China Life Sci 2020;63:1678–87.
[Article] [PubMed] [PMC]31. Wang J, Yuan X. Digestive system symptoms and function in children with COVID-19: a meta-analysis. Medicine (Baltimore) 2021;100:e24897.
[PubMed] [PMC]32. Ashktorab Y, Brim A, Pizuorno A, Gayam V, Nikdel S, Brim H. COVID-19 pediatric patients: gastrointestinal symptoms, presentations, and disparities by race/ethnicity in a large, multicenter US study. Gastroenterology 2021;160:1842–4.
[Article] [PubMed] [PMC]33. Young BE, Ong SWX, Kalimuddin S, Low JG, Tan SY, Loh J, et al. Epidemiologic features and clinical course of patients infected with SARS-CoV-2 in Singapore. JAMA 2020;323:1488–94.
[Article] [PubMed] [PMC]34. Ong SWX, Tan YK, Chia PY, Lee TH, Ng OT, Wong MSY, et al. Air, Surface environmental, and personal protective equipment contamination by severe acute respiratory syndrome coronavirus 2 (SARS-CoV-2) from a symptomatic patient. JAMA 2020;323:1610–2.
[Article] [PubMed] [PMC]35. Xu Y, Li X, Zhu B, Liang H, Fang C, Gong Y, et al. Characteristics of pediatric SARS-CoV-2 infection and potential evidence for persistent fecal viral shedding. Nat Med 2020;26:502–5.
[Article] [PubMed] [PMC]36. Chen N, Zhou M, Dong X, Qu J, Gong F, Han Y, et al. Epidemiological and clinical characteristics of 99 cases of 2019 novel coronavirus pneumonia in Wuhan, China: a descriptive study. Lancet 2020;395:507–13.
[Article] [PubMed] [PMC]37. Götzinger F, Santiago-García B, Noguera-Julián A, Lanaspa M, Lancella L, Calò Carducci FI, et al. COVID-19 in children and adolescents in Europe: a multinational, multicentre cohort study. Lancet Child Adolesc Health 2020;4:653–61.
[PubMed] [PMC]38. Kompaniyets L, Agathis NT, Nelson JM, Preston LE, Ko JY, Belay B, et al. Underlying medical conditions associated with severe COVID-19 illness among children. JAMA Netw Open 2021;4:e2111182.
[Article] [PubMed] [PMC]39. Belay ED, Abrams J, Oster ME, Giovanni J, Pierce T, Meng L, et al. Trends in geographic and temporal distribution of US children with multisystem inflammatory syndrome during the COVID-19 pandemic. JAMA Pediatr 2021;175:837–45.
[Article] [PubMed] [PMC]40. Nyabi O, Bentahir M, Ambroise J, Bearzatto B, Chibani N, Smits B, et al. Diagnostic value of IgM and IgG detection in COVID-19 diagnosis by the mobile laboratory B-LiFE: a massive testing strategy in the piedmont region. Int J Environ Res Public Health 2021;18:3372.
[Article] [PubMed] [PMC]41. Jia X, Zhang P, Tian Y, Wang J, Zeng H, Wang J, et al. Clinical significance of an IgM and IgG test for diagnosis of highly suspected COVID-19. Front Med (Lausanne) 2021;8:569266.
[Article] [PubMed] [PMC]42. Lee SM, Kim IS, Lim S, Lee SJ, Kim WJ, Shin KH, et al. Comparison of serologic response of hospitalized COVID-19 patients using 8 immunoassays. J Korean Med Sci 2021;36:e64.
[Article] [PubMed] [PMC]43. Cevik M, Tate M, Lloyd O, Maraolo AE, Schafers J, Ho A. SARS- CoV-2, SARS-CoV, and MERS-CoV viral load dynamics, duration of viral shedding, and infectiousness: a systematic review and meta-analysis. Lancet Microbe 2021;2:e13–22.
[Article] [PubMed]44. He X, Lau EHY, Wu P, Deng X, Wang J, Hao X, et al. Temporal dynamics in viral shedding and transmissibility of COVID-19. Nat Med 2020;26:672–5.
[Article] [PubMed]45. Cheng HY, Jian SW, Liu DP, Ng TC, Huang WT, Lin HH, et al. Contact tracing assessment of COVID-19 transmission dynamics in taiwan and risk at different exposure periods before and after symptom onset. JAMA Intern Med 2020;180:1156–63.
[Article] [PubMed] [PMC]46. Wang Z, Zhou Q, Wang C, Shi Q, Lu S, Ma Y, et al. Clinical characteristics of children with COVID-19: a rapid review and meta-analysis. Ann Transl Med 2020;8:620.
[Article] [PubMed] [PMC]47. Chen Z, Fu J, Shu Q, Wang W, Chen Y, Hua C, et al. Diagnosis and treatment recommendation for pediatric COVID-19 (the second edition). Zhejiang Da Xue Xue Bao Yi Xue Ban 2020;49:139–46.
[PubMed] [PMC]48. Xu H, Luo Zh, Xu F. Diagnosis and treatment of the 2019 novel coronavirus infection in children. (Trial version 2 of Children's Hospital of Chongqing Medical University). J Pediatr Pharm 2020;(04): Available from: http://kns.cnki.net/kcms/detail/50.1156.R.20200226.1309.002.html.49. Tan L, Wang Q, Zhang D, Ding J, Huang Q, Tang YQ, et al. Lymphopenia predicts disease severity of COVID-19: a descriptive and predictive study. Signal Transduct Target Ther 2020;5:33.
[Article]50. Que Y, Hu C, Wan K, Hu P, Wang R, Luo J, et al. Cytokine release syndrome in COVID-19: a major mechanism of morbidity and mortality. Int Rev Immunol 2022;41:217–30.
[Article] [PubMed] [PMC]51. Mamishi S, Movahedi Z, Mohammadi M, Ziaee V, Khodabandeh M, Abdolsalehi MR, et al. Multisystem inflammatory syndrome associated with SARS-CoV-2 infection in 45 children: a first report from Iran. Epidemiol Infect 2020;148:e196.
[Article] [PubMed] [PMC]52. Zhu N, Zhang D, Wang W, Li X, Yang B, Song J, et al. A novel coronavirus from patients with pneumonia in China, 2019. N Engl J Med 2020;382:727–33.
[Article] [PubMed] [PMC]53. Wu Q, Xing Y, Shi L, Li W, Gao Y, Pan S, et al. Coinfection and other clinical characteristics of COVID-19 in children. Pediatrics 2020;146:e20200961.
[Article] [PubMed]54. Hayıroğlu Mİ, Çınar T, Tekkeşin Aİ. Fibrinogen and D-dimer variances and anticoagulation recommendations in Covid-19: current literature review. Rev Assoc Med Bras (1992) 2020;66:842–8.
[Article] [PubMed]55. Choi KW, Chau TN, Tsang O, Tso E, Chiu MC, Tong WL, et al. Outcomes and prognostic factors in 267 patients with severe acute respiratory syndrome in Hong Kong. Ann Intern Med 2003;139:715–23.
[Article] [PubMed]56. Yu B, Li X, Chen J, Ouyang M, Zhang H, Zhao X, et al. Evaluation of variation in D-dimer levels among COVID-19 and bacterial pneumonia: a retrospective analysis. J Thromb Thrombolysis 2020;50:548–57.
[Article] [PubMed] [PMC]57. Weitz JI, Fredenburgh JC, Eikelboom JW. A test in context: D-Dimer. J Am Coll Cardiol 2017;70:2411–20.
[Article] [PubMed]58. Parri N, Lenge M, Buonsenso D, Coronavirus Infection in Pediatric Emergency Departments (CONFIDENCE) Research Group. Children with Covid-19 in pediatric emergency departments in Italy. N Engl J Med 2020;383:187–90.
[Article] [PubMed] [PMC]59. Lu X, Zhang L, Du H, Zhang J, Li YY, Qu J, et al. SARS-CoV-2 infection in children. N Engl J Med 2020;382:1663–5.
[PubMed]60. Ahmed J, Rizwan T, Malik F, Akhter R, Malik M, Ahmad J, et al. COVID-19 and liver injury: a systematic review and meta-analysis. Cureus 2020;12:e9424.
[Article] [PubMed] [PMC]61. Guan WJ, Ni ZY, Hu Y, Liang WH, Ou CQ, He JX, et al. Clinical characteristics of coronavirus disease 2019 in China. N Engl J Med 2020;382:1708–20.
[PubMed]62. Fan H, Cai J, Tian A, Li Y, Yuan H, Jiang Z, et al. Comparison of liver biomarkers in 288 COVID-19 patients: a mono-centric study in the early phase of pandemic. Front Med (Lausanne) 2021;7:584888.
[Article] [PubMed] [PMC]63. Cheng Y, Luo R, Wang K, Zhang M, Wang Z, Dong L, et al. Kidney disease is associated with in-hospital death of patients with COVID-19. Kidney Int 2020;97:829–38.
[Article] [PubMed] [PMC]64. Sorkhi H, Esmaeili Dooki M, Nikpour M, Mohammadi M, Mohammadpour-Mir A, Kiani M, et al. COVID-19 and renal involvement in children: a retrospective study. Caspian J Intern Med 2022;13(Suppl 3): 193–8.
[PubMed] [PMC]65. Oterino Serrano C, Alonso E, Andrés M, Buitrago NM, Pérez Vigara A, Parrón Pajares M, et al. Pediatric chest x-ray in covid-19 infection. Eur J Radiol 2020;131:109236.
[Article] [PubMed] [PMC]66. Kumar J, Meena J, Yadav A, Yadav J. Radiological findings of COVID-19 in children: a systematic review and meta-analysis. J Trop Pediatr 2021;67:fmaa045.
[Article] [PubMed] [PMC]67. Udomsinprasert W, Jittikoon J, Sangroongruangsri S, Chaikledkaew U. Circulating levels of interleukin-6 and interleukin-10, but not tumor necrosis factor-alpha, as potential biomarkers of severity and mortality for COVID-19: systematic review with meta-analysis. J Clin Immunol 2021;41:11–22.
[Article] [PubMed] [PMC]68. Wilson JG, Simpson LJ, Ferreira AM, Rustagi A, Roque J, Asuni A, et al. Cytokine profile in plasma of severe COVID-19 does not differ from ARDS and sepsis. JCI Insight 2020;5:e140289.
[Article] [PubMed] [PMC]69. Alabd S, Yameny A. The association between tumor necrosis factor-alpha level (TNF-α) and moderate COVID-19 patients in Egypt. J Biosci Appl Res 2021;7:223–8.
[Article]70. Han H, Ma Q, Li C, Liu R, Zhao L, Wang W, et al. Profiling serum cytokines in COVID-19 patients reveals IL-6 and IL-10 are disease severity predictors. Emerg Microbes Infect 2020;9:1123–30.
[Article] [PubMed] [PMC]71. Bani Hani A, Abu Tarboush N, Bani Ali M, Alabhoul F, Alansari F, Abuhani A, et al. Serum ACE2 level is associated with severe SARS-CoV-2 infection: a cross-sectional observational study. Biomark Insights 2022;17:11772719221125123.
[PubMed] [PMC]72. Saleh A, Sultan A, Elashry MA, Farag A, Mortada MI, Ghannam MA, et al. Association of TNF-α G-308 a promoter polymorphism with the course and outcome of COVID-19 patients. Immunol Invest 2022;51:546–57.
[Article] [PubMed] [PMC]73. Sotomayor-Lugo F, Alemañy-Díaz Perera C, Roblejo-Balbuena H, Zúñiga-Rosales Y, Monzón-Benítez G, Suárez-Besil B, et al. The role of tumor necrosis factor alpha -308A>G polymorphism on the clinical states of SARS-CoV-2 infection. Egypt J Med Hum Genet 2022;23:55.
[Article] [PubMed] [PMC]74. Chen YY, Zhang P, Zhou XM, Liu D, Zhong JC, Zhang CJ, et al. Relationship between genetic variants of ACE2 gene and circulating levels of ACE2 and its metabolites. J Clin Pharm Ther 2018;43:189–95.
[Article] [PubMed]75. Liu D, Chen Y, Zhang P, Zhong J, Jin L, Zhang C, et al. Association between circulating levels of ACE2-Ang-(1-7)-MAS axis and ACE2 gene polymorphisms in hypertensive patients. Medicine (Baltimore) 2016;95:e3876.
[Article] [PubMed] [PMC]76. Möhlendick B, Schönfelder K, Breuckmann K, Elsner C, Babel N, Balfanz P, et al. ACE2 polymorphism and susceptibility for SARS-CoV-2 infection and severity of COVID-19. Pharmacogenet Genomics 2021;31:165–71.
[Article]77. Ng DC, Liew CH, Tan KK, Chin L, Ting GSS, Fadzilah NF, et al. Risk factors for disease severity among children with Covid-19: a clinical prediction model. BMC Infect Dis 2023;23:398.
[Article] [PubMed] [PMC]78. Zhou B, Yuan Y, Wang S, Zhang Z, Yang M, Deng X, et al. Risk profiles of severe illness in children with COVID-19: a meta-analysis of individual patients. Pediatr Res 2021;90:347–52.
[Article] [PubMed] [PMC]79. Graff K, Smith C, Silveira L, Jung S, Curran-Hays S, Jarjour J, et al. Risk factors for severe COVID-19 in children. Pediatr Infect Dis J 2021;40:e137–45.
[Article]80. Fernandes DM, Oliveira CR, Guerguis S, Eisenberg R, Choi J, Kim M, et al. Severe acute respiratory syndrome coronavirus 2 clinical syndromes and predictors of disease severity in hospitalized children and youth. J Pediatr 2021;230:23. –31. e10.
[Article] [PubMed] [PMC]81. Wong JJM, Abbas Q, Chuah SL, Malisie RF, Pon KM, Katsuta T, et al. Comparative analysis of pediatric COVID-19 infection in Southeast Asia, South Asia, Japan, and China. Am J Trop Med Hyg 2021;105:413–20.
[Article] [PubMed] [PMC]82. Guo CX, He L, Yin JY, Meng XG, Tan W, Yang GP, et al. Epidemiological and clinical features of pediatric COVID-19. BMC Med 2020;18:250.
[Article] [PubMed] [PMC]83. Çetin C, Karaaslan A, Akın Y, Arifoglu M, Rona G, Demirhan R. Relationship of chest computed tomography score with disease severity and laboratory values in children with COVID-19. J Paediatr Child Health 2022;58:802–8.
[PubMed]84. Soltani J, Sedighi I, Shalchi Z, Sami G, Moradveisi B, Nahidi S. Pediatric coronavirus disease 2019 (COVID-19): an insight from west of Iran. North Clin Istanb 2020;7:284–91.
[Article] [PubMed] [PMC]85. Kiani M, Mohammadpour-Mir A, Sorkhi H, Esmaeili-dooki M, Nikpour M, Babazadeh K, et al. Multi-organ presentation of children with COVID-19 infection in the North of Iran: a retrospective study. Int J Pediatr 2021;9:13411–9.86. Smith C, Odd D, Harwood R, Ward J, Linney M, Clark M, et al. Deaths in children and young people in England after SARS-CoV-2 infection during the first pandemic year. Nat Med 2022;28:185–92.
[Article] [PubMed]87. Bhopal SS, Bagaria J, Olabi B, Bhopal R. Children and young people remain at low risk of COVID-19 mortality. Lancet Child Adolesc Health 2021;5:e12–3.
[Article] [PubMed] [PMC]88. Tan L, Ganapathy SS, Chan YM, Alias N, Nasaruddin NH, Khaw WF, et al. Estimating the COVID-19 mortality burden over two full years of the pandemic in Malaysia. Lancet Reg Health West Pac 2022;22:100456.
[Article] [PubMed] [PMC]89. Ruan Q, Yang K, Wang W, Jiang L, Song J. Clinical predictors of mortality due to COVID-19 based on an analysis of data of 150 patients from Wuhan, China. Intensive Care Med 2020;46:846–8.
[Article] [PubMed] [PMC]